Article first published 12th October 2021, updated 3rd July 2024. The optical transceiver market is…
Article first published 12th October 2021, updated 3rd July 2024.
The optical transceiver market is expected to double in size by 2025, and coherent optical technology has come a long way over the past decade to become more accessible and thus have a greater impact on this market. When Nortel (later Ciena) introduced the first commercial coherent transponder in 2008, the device was a bulky, expensive line card with discrete components distributed on multiple circuit boards.
As time went by, coherent devices got smaller and consumed less power. By 2018, most coherent line card transponder functions could be miniaturized into CFP2 transceiver modules that were the size of a pack of cards and could plug into modules with pluggable line sides. QSFP modules followed a couple of years later, and they were essentially the size of a large USB stick and could be plugged directly into routers. They were a great fit for network operators who wanted to take advantage of the lower power consumption and cost, field replaceability, vendor interoperability, and pay-as-you-grow features.
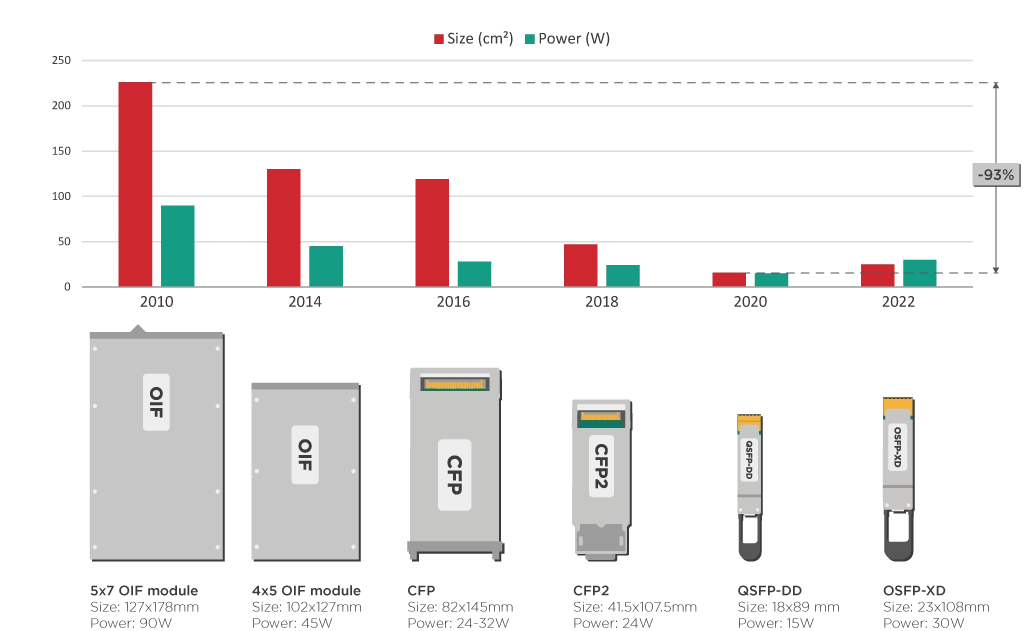
Despite the onset of pluggables, the big, proprietary line card optical engines have still played a role in the market by focusing on delivering best-in-class optical performance. The low-noise, high-power signals they produce have the longest reach for optical links and have wider compatibility with the ROADM multiplexers used in metro and long-haul networks. The smaller CFP2 modules produce, at best, roughly half the laser power of the line card modules, which limits their reach. Meanwhile, even smaller QSFP form factors can not fit optical amplifier components, so their transmit power and reach are much more limited than even a CFP2 module.
All in all, the trade-offs were clear: go for proprietary line card transponders if you want best-in-class performance and longest reach, and go for CFP2 or QSFP transceivers if you want a smaller footprint and power consumption. This trade-off, however, limits the more widespread adoption of coherent technology. For example, mobile network operators need high performance, a smaller footprint, and power consumption so that their metro and access networks can meet the rising demands for 5G data.
So what if we told you the current paradigm of line card transponders versus pluggable transceivers is outdated? Recent improvements in electronic and photonic integration have squeezed more performance and functions into smaller form factors, allowing pluggable devices to almost catch up to line cards.
Integration Enables Line Card Performance in a Pluggable Form Factor
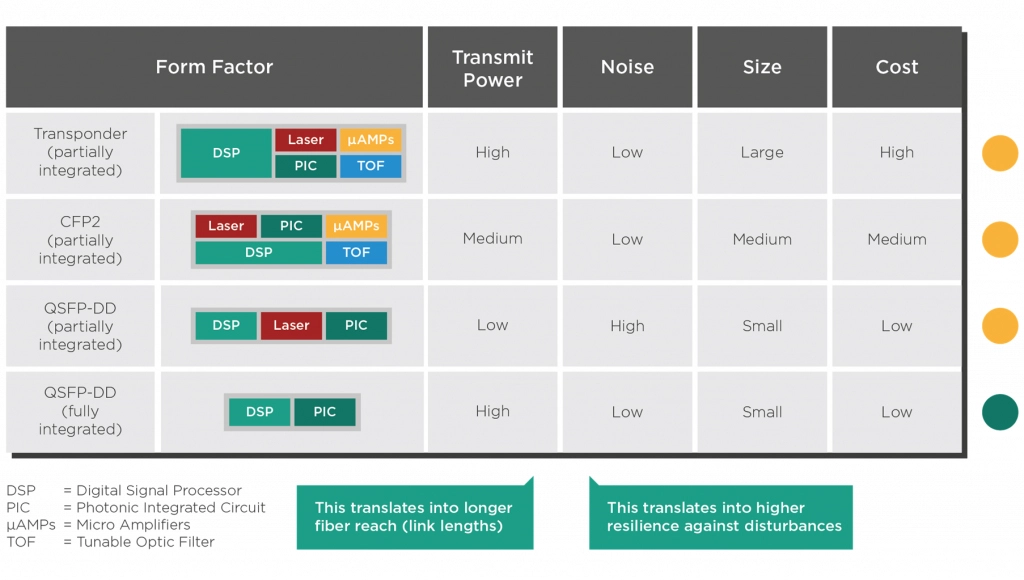
The advances in photonic integration change the game and can enable high performance and transmit power in the smallest pluggable transceiver form factors. By integrating all photonic functions on a single chip, including lasers and optical amplifiers, pluggable transceiver modules can achieve transmit power levels closer to those of line card transponder modules while still keeping the smaller QSFP router pluggable form factor, power consumption, and cost.
Full photonic integration increases the transmit power further by minimizing the optical losses due to the use of more efficient optical modulators, fewer coupling losses compared to silicon, and the integration of the laser device on the same chip as the rest of the optical components.
Modern ASICs Can Fit Electronics Functions in a Pluggable Form Factor
As important as optical performance is, though, pluggable transceivers also needed improvements on the electronic side. Traditionally, line card systems not only had better optical performance but also broader and more advanced electronic functionalities, such as digital signal processing (DSP), advanced forward error correction (FEC), encryption, and advanced modulation schemes. These features are usually implemented on electronic application-specific integrated circuits (ASICs).
ASICs benefit from the same CMOS process improvements that drive progress in consumer electronics. Each new CMOS process generation can fit more transistors into a single chip. Ten years ago, an ASIC for line cards had tens of millions of transistors, while the 7nm ASIC technology used in modern pluggables has more than five billion transistors. This progress in transistor density allows ASICs to integrate more electronic functions than ever into a single chip while still making the chip smaller. Previously, every function—signal processing, analog/digital conversion, error correction, multiplexing, encryption—required a separate ASIC, but now they can all be consolidated on a single chip that fits in a pluggable transceiver.
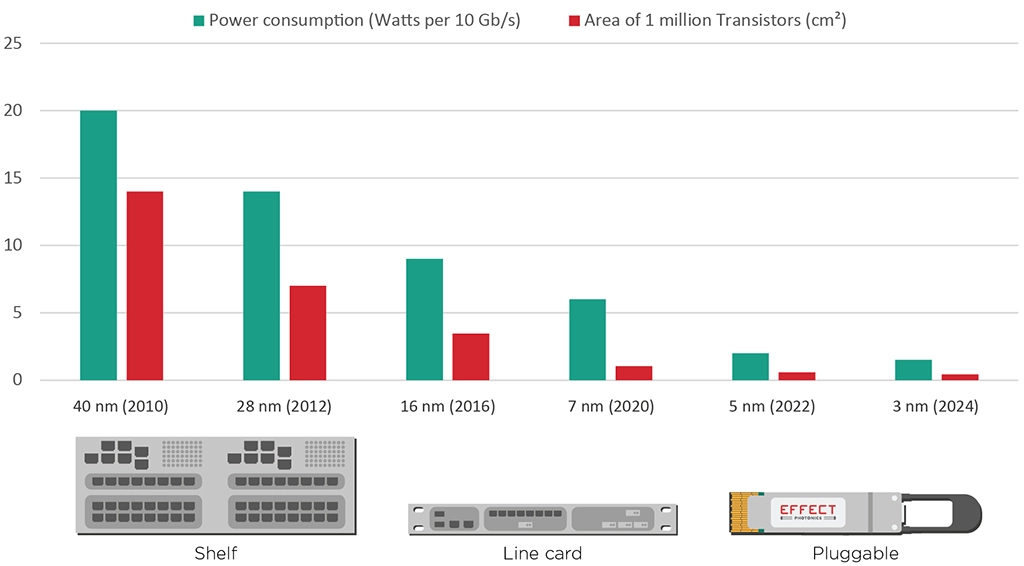
This increase in transistor density and integration also leads to massive gains in power consumption and performance. For example, modern transceivers using 7nm ASICs have decreased their consumption by 50% compared to the previous generation using 16nm ASICs while delivering roughly a 30% increase in bandwidth and baud rates. By 2022, ASICs in pluggables will benefit from a newer 5nm CMOS process, enabling further improvements in transistor density, power consumption, and speed.
Electronic Integration Enables Line-Card System Management in a Pluggable Form Factor
The advancements in CMOS technology also enable the integration of system-level functions into a pluggable transceiver. Previously, functions such as in-band network management and security, remote management, autotuneability, or topology awareness had to live on the shelf controller or in the line card interface, but that’s not the case anymore. Thanks to the advances in electronic integration, we are closer than ever to achieving a full, open transponder on a pluggable that operates as part of the optical network. These programmable, pluggable transceivers provide more flexibility than ever to manage access networks.
For example, the pluggable transceiver could run in a mode that prioritizes high-performance or one that prioritizes low consumption by using simpler and less power-hungry signal processing and error correction features. Therefore, these pluggables could provide high-end performance in the smallest form-factor or low and mid-range performance at lower power consumption than embedded line card transponders.
EFFECT Photonics has already started implementing these system-management features in its products. For example, our direct-detect SFP+ transceiver modules feature NarroWave technology, which allows customers to monitor and control remote SFP+ modules from the central office without making any hardware or software changes in the field. NarroWave is agnostic of vendor equipment, data rate, or protocol of the in-band traffic.
Pluggable transceivers also provide the flexibility of multi-vendor interoperability. High-performance line card transponders have often prioritized using proprietary features to increase performance while neglecting interoperability. The new generations of pluggables don’t need to make this trade-off: they can operate in standards-compatible modes for interoperability or in high-performance modes that use proprietary features.
Takeaways
Coherent technology was originally reserved for premium long-distance links where performance is everything. Edge and access networks could not use this higher-performance technology since it was too bulky and expensive.
Photonic integration technology like the one used by EFFECT Photonics helps bring these big, proprietary, and expensive line card systems into a router pluggable form factor. This tech has squeezed more performance into a smaller area and at lower power consumption, making the device more cost-effective. Combining the improvements in photonic integration with the advances in electronic integration for ASICs, the goal of having a fully programmable transponder in a pluggable is practically a reality. Photonic integration will be a disruptive technology that will simplify network design and operation and reduce network operators’ capital and operating expenses.
The impact of this technological improvement in pluggable transceivers was summarized deftly by Keven Wollenweber, VP of Product Management for Cisco’s Routing Portfolio:
“Technology advancements have reached a point where coherent pluggables match the QSFP-DD form factor of grey optics, enabling a change in the way our customers build networks. 100G edge and access optimized coherent pluggables will not only provide operational simplicity, but also scalability, making access networks more future proof.”
Tags: 100G, access network, ASIC, CFP, coherent optics, CoherentPIC, DSP, edge network, electronic integration, fully integrated, Fully Integrated PICs, Integrated Photonics, line card, metro access, miniaturization, NarroWave, optical transceivers, photonic integration, PIC, pluggable, pluggable transceiver, QSFP, SFP, small form factor, sustainability telecommunication