The Future of Coherent Transceivers in the Access
The demand for data and other digital services is rising exponentially. From 2010 to 2020, Internet users worldwide doubled, and global internet traffic increased 12-fold. From 2020 to 2026, internet traffic will likely increase 5-fold. To meet this demand, datacom and telecom operators need constantly upgrade their transport networks.
The major obstacles in this upgrade path remain the power consumption, thermal management, and affordability of transceivers. Over the last two decades, power ratings for pluggable modules have increased as we moved from direct detection to more power-hungry coherent transmission: from 2W for SFP modules to 3.5 W for QSFP modules and now to 14W for QSSFP-DD and 21.1W for OSFP form factors. This power consumption increase seems incompatible with the power constraints of the network edge.
This article will review trends in data rate, power consumption, and footprint for transceivers in the network edge that aim to address these challenges.
Downscaling Data Rates for the Access
Given the success of 400ZR pluggable coherent solutions in the market, discussions in the telecom sector about a future beyond 400G pluggables have often focused on 800G solutions and 800ZR. However, there is also increasing excitement about “downscaling” to 100G coherent products for applications in the network edge.
In the coming years, 100G coherent uplinks will become increasingly widespread in deployments and applications throughout the network edge. Some mobile access networks use cases must upgrade their existing 10G DWDM link aggregation into a single coherent 100G DWDM uplink. Meanwhile, cable networks and business services are upgrading their customer links from 1Gbps to 10Gbps, and this migration will be the significant factor that will increase the demand for coherent 100G uplinks. For carriers who provide converged cable/mobile access, these upgrades to 100G uplinks will enable opportunities to overlay more business services and mobile traffic into their existing cable networks.
You can read more about these developments in our previous article, When Will the Network Edge Go Coherent?
Moving Towards Low Power
Data centers and 5G networks might be hot commodities, but the infrastructure that enables them runs even hotter. Electronic equipment generates plenty of heat; the more heat energy an electronic device dissipates, the more money and energy must be spent to cool it down. These power efficiency issues do not just affect the environment but also the bottom lines of communications companies.
As shown in the table below, the growth of data centers and wireless networks will continue to drive power consumption upwards.
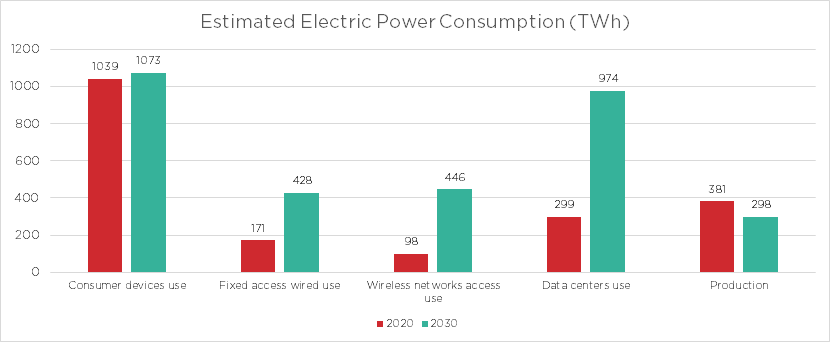
These power constraints are even more pressing in the access network sector. Unlike data centers and the network core, access network equipment lives in uncontrolled environments with limited cooling capabilities. Therefore, every extra watt of pluggable power consumption will impact how vendors and operators design their cabinets and equipment.
These struggles are a major reason why QSFP28 form factor solutions are becoming increasingly attractive in the 100ZR domain. Their power consumption (up to 6 watts) is lower than that of QSFP-DD form factors (up to 14 Watts), which allows them to be stacked more densely in access network equipment rooms. Besides, QSFP28 modules are compatible with existing access network equipment, which often features QSFP28 slots.
Aside from the move to QSFP28 form factors for 100G coherent, EFFECT Photonics also believes in two other ways to reduce power consumption.
- Increased Integration: The interconnections among smaller, highly-integrated optical components consume less power than those among more discrete components. We will discuss this further in the next section.
- Co-Design: As we explained in a previous article about fit-for-platform DSPs, a transceiver optical engine designed on the indium phosphide platform could be designed to run at a voltage compatible with the DSP’s signal output. This way, the optimized DSP could drive the PIC directly without needing a separate analog driver, doing away with a significant power conversion overhead
Can We Still Move Towards Smaller Footprints?
Moving toward smaller pluggable footprints should not necessarily be a goal, but as we mentioned in the previous section, it is a means toward the goal of lower power consumption. Decreasing the size of optical components and their interconnections means that the light inside the chip will travel a smaller distance and accumulate fewer optical losses.
Let’s look at an example of lasers. In the last decade, technological progress in tunable laser packaging and integration has matched the need for smaller footprints. In 2011, tunable lasers followed the multi-source agreement (MSA) for integrable tunable laser assemblies (ITLAs). The ITLA package measured around 30.5 mm in width and 74 mm in length. By 2015, tunable lasers were sold in the more compact Micro-ITLA form factor, which cut the original ITLA package size in half. And in 2019, laser developers (see examples here and here) announced a new Nano-ITLA form factor that reduced the size by almost half again.
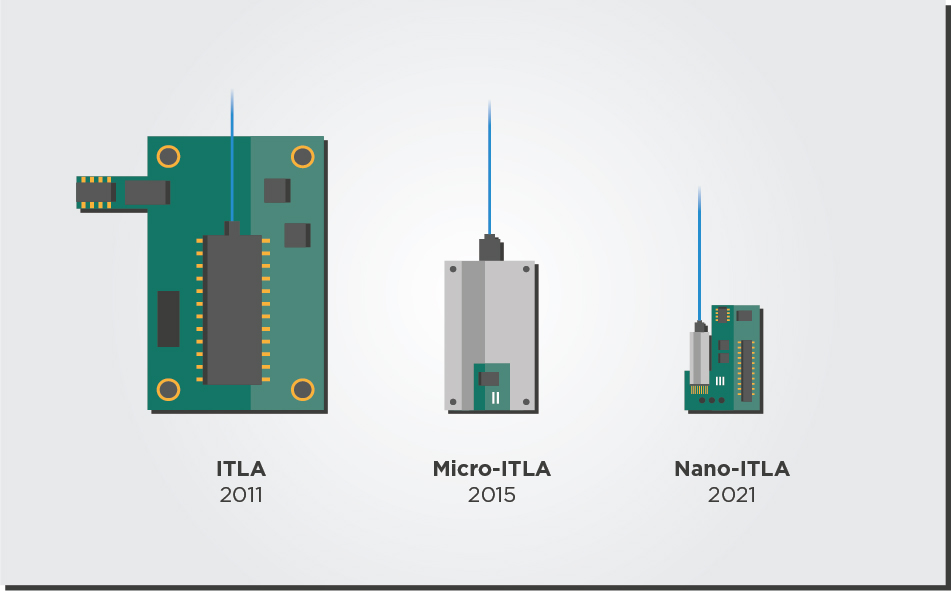
Reducing the footprint of tunable lasers in the future will need even greater integration of their parts. For example, every tunable laser needs a wavelength locker component that can stabilize the laser’s output regardless of environmental conditions such as temperature. Integrating the wavelength locker component on the laser chip instead of attaching it externally would help reduce the laser package’s footprint and power consumption.
Another potential future to reduce the size of tunable laser packages is related to the control electronics. The current ITLA standards include the complete control electronics on the laser package, including power conversion and temperature control. However, if the transceiver’s main board handles some of these electronic functions instead of the laser package, the size of the laser package can be reduced.
This approach means the reduced laser package would only have full functionality if connected to the main transceiver board. However, some transceiver developers will appreciate the laser package reduction and the extra freedom to provide their own laser control electronics.
Takeaways
The ever-increasing bandwidth demands in access networks force coherent pluggables to face the complex problem of maintaining a good enough performance while moving to lower cost and power consumption.
The move towards 100G coherent solutions in QSFP28 form factors will play a major role in meeting the power requirements of the access network sector. Further gains can be achieved with greater integration of optical components and co-designing the optics and electronic engines of the transceiver to reduce inefficiencies. Further gains in footprint for transceivers can also be obtained by eliminating redundant laser control functions in both the laser package and the main transceiver board.