Shining a Light on Four Tunable Lasers
The world is moving towards tunability. Datacom and telecom companies may increase their network capacity without investing in new fiber infrastructure thanks to tunable lasers and dense wavelength division multiplexing (DWDM). Furthermore, the miniaturization of coherent technology into pluggable transceiver modules has enabled the widespread implementation of IP over DWDM solutions. Self-tuning algorithms have also contributed to the broad adoption of DWDM systems since they reduce the complexity of deployment and maintenance.
The tunable laser is a core component of all these tunable communication systems, both direct detection and coherent. The fundamental components of a laser are the following:
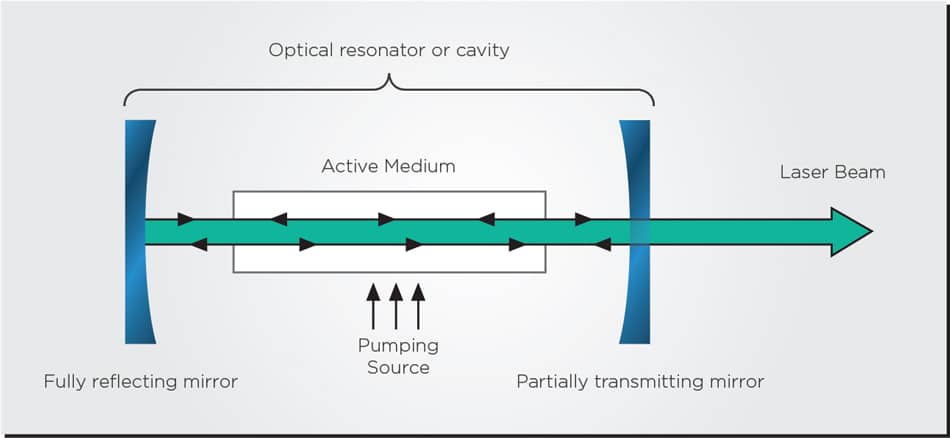
- An optical resonator (also called an optical cavity) that allows laser light to re-circulate and feed itself back. Resonators can be linear or ring-shaped. Linear resonators have a highly reflective mirror on one end and a partially-reflective mirror on the other, which acts as a coupler that lets the laser light out. On the other hand, ring resonators use a waveguide as an output coupler.
- An active medium (also called a gain medium) inside the resonator that, when pumped by an external energy source, will amplify the power of light by a process called stimulated emission.
- A pump source is the external energy source that powers the amplification process of the gain medium. The typical tunable laser used in communications will use an electrical pump, but some lasers can also use an optical pump (i.e., another light source).
As light circulates throughout the resonator, it passes multiple times through the pumped gain medium, amplifying itself and building up power to become the highly concentrated and coherent beam of light we know as a laser.
There are multiple ways to tune lasers, but let’s discuss three common tuning methods. These methods can and are often used together.
- Tuning the Gain Medium: By changing the pump intensity or environmental conditions such as its temperature, the gain medium can amplify different frequencies of light.
- Tuning the Resonator Length: The light inside a resonator goes back and forth at a frequency that depends on the length of the resonator. So making the resonator shorter or longer can change its frequency.
- Tuning by Filtering: Adding a filtering element inside or outside the resonator, such as a diffraction grating (i.e., a periodic mirror), allows the laser to “select” a specific frequency.
With this short intro on how lasers work and can be tuned, let’s dive into some of the different tunable lasers used in communication systems.
Distributed Feedback Lasers
Distributed Feedback (DFB) lasers are unique because they directly etch a grating onto the gain medium. This grating acts as a periodic mirror, forming the optical resonator needed to recirculate light and create a laser beam. These lasers are tunable by tuning the temperature of the gain medium and by filtering with the embedded grating.
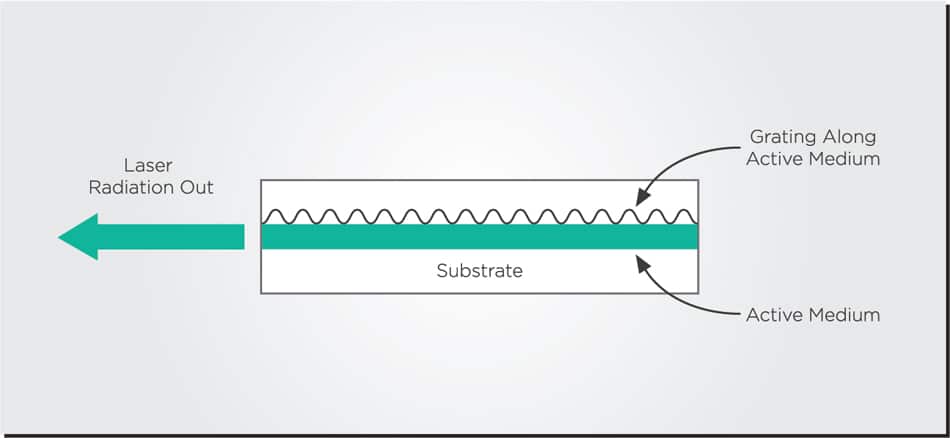
Compared to their predecessors, DFB lasers could produce very pure, high-quality laser light with lower complexity in design and manufacturing that could be easily integrated into optical fiber systems. These characteristics benefited the telecommunications sector, which needed lasers with high purity and low noise that could be produced at scale. After all, the more pure (i.e., lower linewidth) a laser is, the more information it can encode. Thus, DFB lasers became the industry’s solution for many years.
The drawback of DFB lasers is that embedding the grating element in the gain medium makes them more sensitive and unstable. This sensitivity narrows their tuning range and makes them less reliable as they age.
Distributed Bragg Reflector (DBR) Lasers
A simple way to improve the reliability compared to a DFB laser is to etch the grating element outside the gain medium instead of inside. This grating element (which in this case is called a Bragg reflector) acts as a mirror that creates the optical resonator and amplifies the light inside. This setup is called a distributed Bragg reflector (DBR) laser.
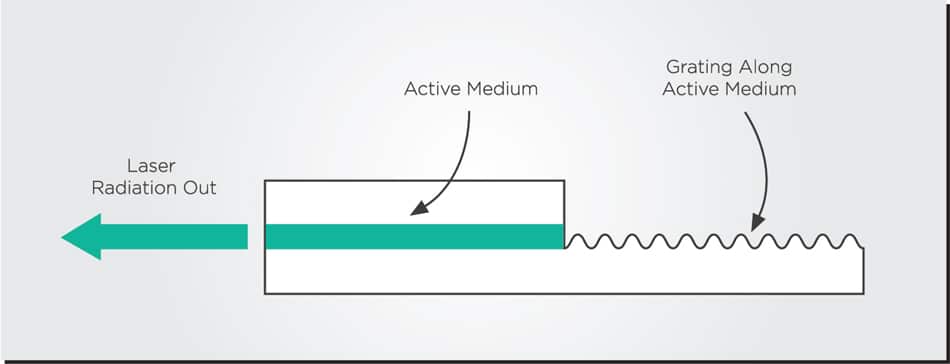
While, in principle, a DBR laser does not have a wider tuning range than a DFB laser, its tuning behavior is more reliable over time. Since the grating is outside the gain medium, the DBR laser is less sensitive to environmental fluctuations and more reliable as it ages. However, as coherent and DWDM systems became increasingly important, the industry needed a greater tuning range that DFB and DBR lasers alone could not provide.
External Cavity Lasers (ECL)
Interestingly enough, one of the most straightforward ways to improve the quality and tunability of a semiconductor laser is to use it inside a second, somewhat larger resonator. This setup is called an external cavity laser (ECL) since this new resonator or cavity will use additional optical elements external to the original laser.
The main modification to the original semiconductor laser is that instead of having a partially reflective mirror as an output coupler, the coupler will use an anti-reflection coating to become transparent. This helps the original laser resonator capture more light from the external cavity.
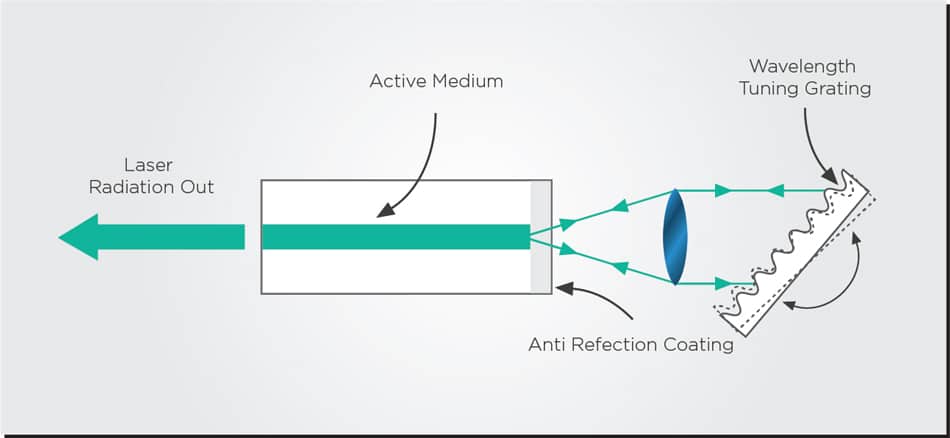
The new external resonator provides more degrees of freedom for tuning the laser. If the resonator uses a mirror, then the laser can be tuned by moving the mirror a bit and changing the length of the resonator. If the resonator uses a grating, it has an additional element to tune the laser by filtering.
ECLs have become the state-of-the-art solution in the telecom industry: they use a DFB or DBR laser as the “base laser” and external gratings as their filtering element for additional tuning. These lasers can provide a high-quality laser beam with low noise, narrow linewidth, and a wide tuning range. However, they came with a cost: manufacturing complexity.
ECLs initially required free-space bulk optical elements, such as lenses and mirrors, for the external cavity. One of the hardest things to do in photonics is coupling between free-space optics and a chip. This alignment of the free-space external cavity with the original laser chip is extremely sensitive to environmental disturbances. Therefore, their coupling is often inefficient and complicates manufacturing and assembly processes, making them much harder to scale in volume.
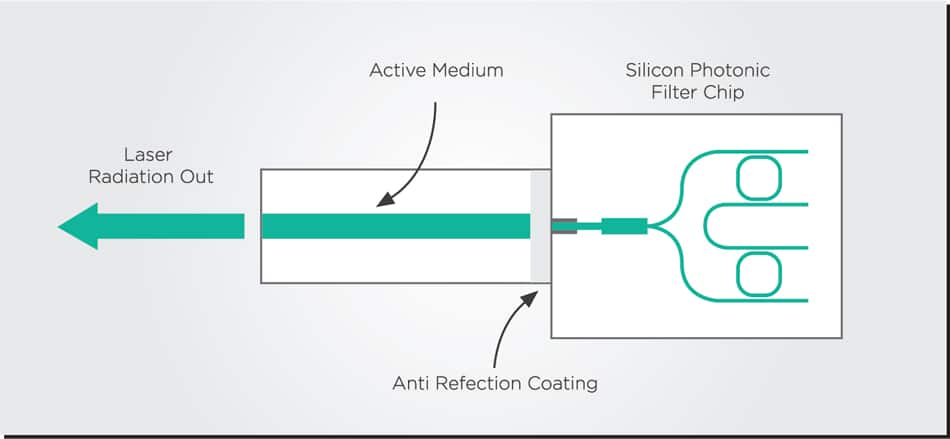
Laser developers have tried to overcome this obstacle by manufacturing the external cavity on a separate chip coupled to the original laser chip. Coupling these two chips together is still a complex problem for manufacturing but more feasible and scalable than coupling from chip to free space optics. This is the direction many major tunable laser developers will take in their future products.
Integrated Tunable Ring Lasers
As we explained in the introductory section, linear resonators are those in which light bounces back and forth between two mirrors. However, ring resonators take a different approach to feedback: the light loops multiple times inside a ring that contains the active medium. The ring is coupled to the rest of the optical circuit via a waveguide.
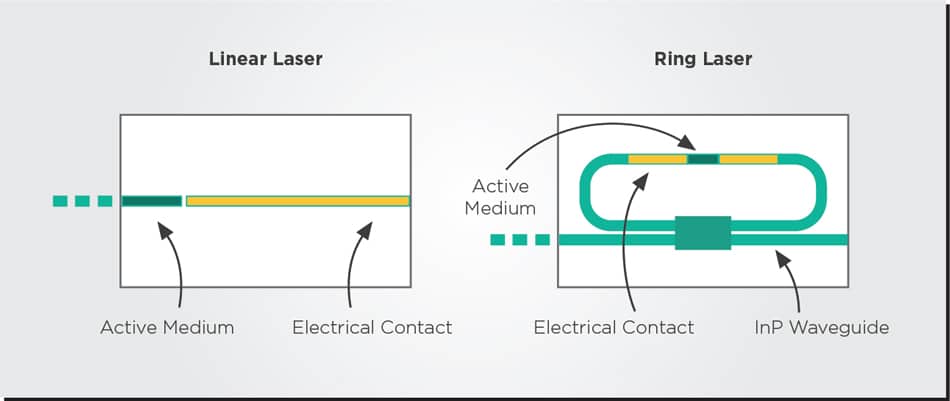
The power of the ring resonator lies in its compactness, flexibility, and integrability. While a single ring resonator is not that impressive or tunable, using multiple rings and other optical elements allows them to achieve performance and tunability on par with the state-of-the-art tunable lasers that use linear resonators.
Most importantly, these widely tunable ring lasers can be entirely constructed on a single chip of Indium Phosphide (InP) material. As shown in this paper from the Eindhoven University of Technology, these lasers can even be built with the same basic building blocks and processes used to make other elements in the InP photonic integrated circuit (PIC).
This high integration of ring lasers has many positive effects. It can avoid inefficient couplings and make the laser more energy efficient. Furthermore, it enables the development of a monolithically integrated laser module where every element is included on the same chip. This includes integrating the wavelength locker component on the same chip, an element most state-of-the-art lasers attach separately.
As we have argued in previous articles, the more elements can be integrated into a single chip, the more scalable the manufacturing process can become.
Takeaways
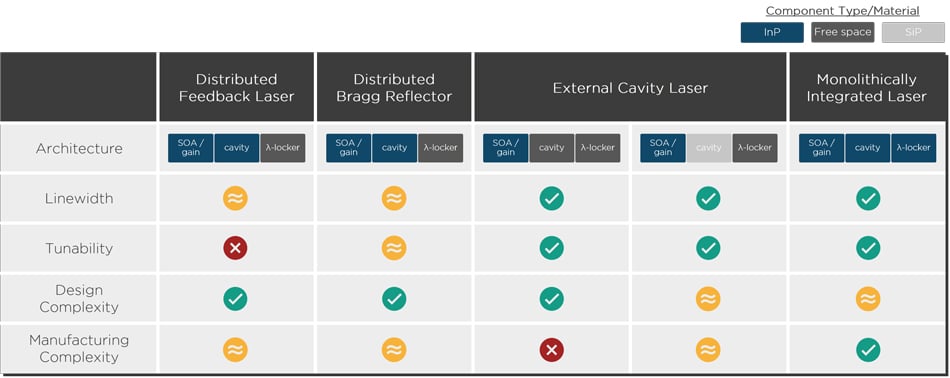
Factors such as output power, noise, linewidth, tuning range, and manufacturability are vital when deciding which kind of laser to use. A DFB or DBR laser should do the job if wide tunability is not required. Greater tuning range will require an external cavity laser, but if the device must be manufactured at a large volume, an external cavity made on a chip instead of free-space optics will scale more easily. The latter is the tunable laser solution the telecom industry is gravitating towards.
That being said, ring lasers are a promising alternative because they can enable a widely tunable and monolithically integrated laser with all elements, including wavelength locker, on the same chip. This setup is ideal for scaling into high production volumes.