Reconfigurable DSPs for Versatile Pluggables
Carriers must solve the dilemma of how to use small and affordable coherent pluggables while still having enough performance and reach to cover most of their network links. At EFFECT Photonics, we believe coherent pluggables with an optical System-on-Chip (SoC) can play a vital role in solving this carrier’s dilemma and simplifying network upgrades. Meanwhile, these new coherent pluggables could also enable longer, and higher-capacity data center interconnects for more sustainable data center architectures.
Another big factor in making networks more affordable and sustainable is software-defined networks (SDNs) and automation. SDNs enable the network function virtualization (NFV), which allows operators to implement more functions to manage and orchestrate the network. Operators will reap even further benefits by using an artificial intelligence (AI) management and orchestration layer with the SDN/NFV layer, as shown in the figure below.
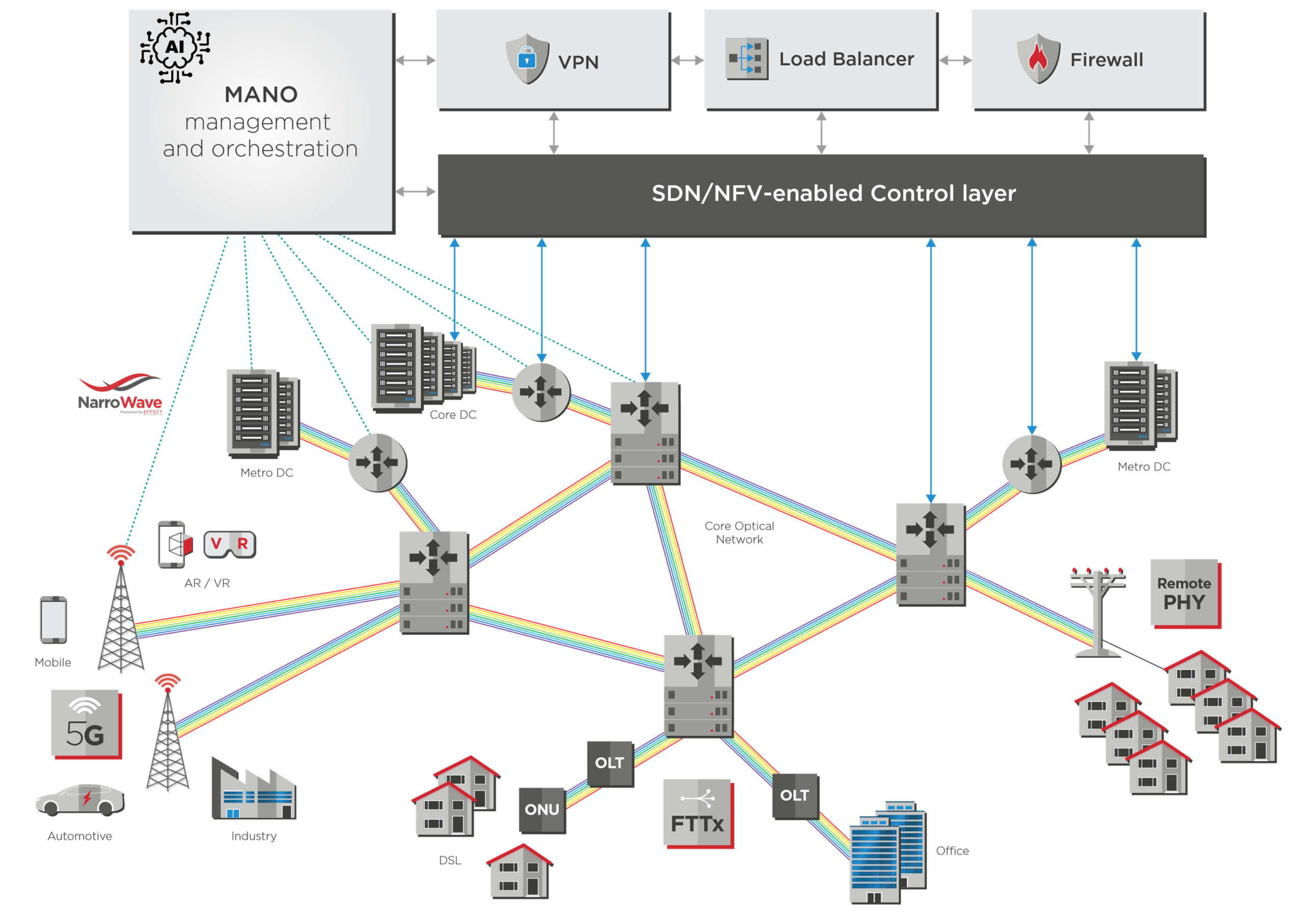
However, creating a fully automated network that can handle the ever-increasing amount of data and connected devices requires us to go beyond just adjusting the higher network layers. The orchestration and management software should also interface with the physical layer of the network, and that requires smart optical pluggable that adapt to varying network requirements. These requirements place additional burdens on digital signal processors (DSPs). DSPs are a vital component of coherent communication systems, as they perform the coding, decoding, and error corrections on the optical signal. Fortunately, the advances in electronic integration and standards make it so that a versatile coherent pluggable can benefit from an equally versatile and reconfigurable DSP.
Future Automated Networks Must Also Work on the Physical Layer
Telecom and datacom providers who want to become market leaders must scale up while also learning to allocate their existing network resources most efficiently and dynamically. SDNs can help achieve this efficient, dynamic network management. In a nutshell, the SDN paradigm separates the switching hardware from the software, allowing operators to virtualize network functions in a single centralized controller unit. This centralized management and orchestration (MANO) layer can implement network functions that the switches do not, allowing network operators to allocate network resources more intelligently and dynamically. This added flexibility and optimization will improve network outcomes for operators.
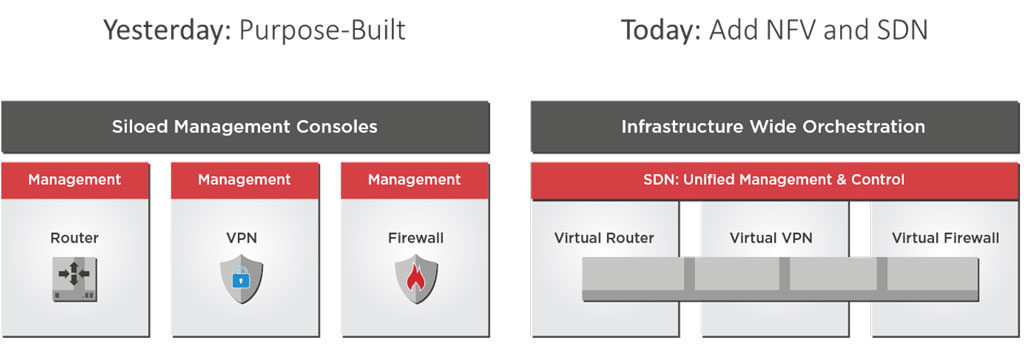
However, the upcoming 5G networks will consist of a massive number of devices, software applications, and technologies. EFFECT Photonics believes that handling all these new devices and use cases will require self-managed, zero-touch automated networks. Realizing this full network automation requires two additional components alongside SDN and NFV:
- Artificial intelligence and machine learning algorithms for complete network automation: For example, AI in network management will become a significant factor in reducing the energy consumption of future telecom networks.
- Sensor and control data flow across all OSI model layers, including the physical layer: As networks get bigger and more complex, the management and orchestration (MANO) software needs more degrees of freedom and knobs to adjust. Next-generation MANO software needs to adjust and optimize both the physical and network layers to fit the network best.
Achieving the second goal requires smart optical equipment and components that can be diagnosed and managed remotely from the MANO layer. This context is where smart pluggable transceivers with reconfigurable DSPs come into play.
The Importance of Standardized Error Correction
Forward error correction (FEC) implemented by DSPs has become a vital component of coherent communication systems. FEC makes the coherent link much more tolerant to noise than a direct detect system and enables much longer reach and higher capacity. Thanks to FEC, coherent links can handle bit error rates that are literally a million times higher than a typical direct detect link. In other words, FEC algorithms allow the DSP to enhance the link performance without changing the hardware. This enhancement is analogous to imaging cameras: image processing algorithms allow the lenses inside your phone camera to produce a higher-quality image.
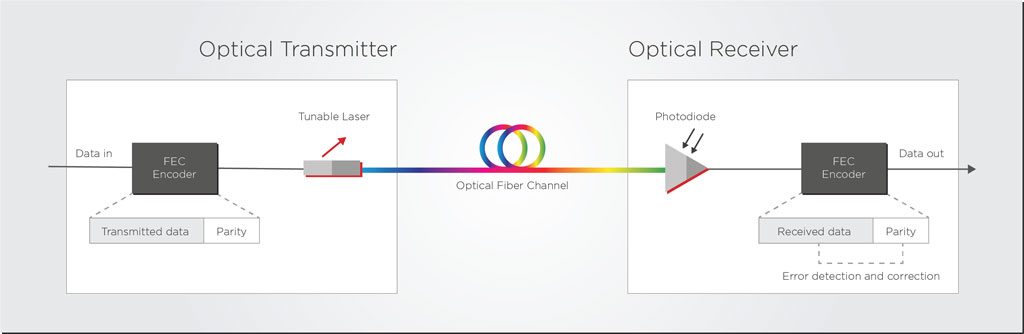
When coherent transmission emerged, all FEC algorithms were proprietary. Equipment and component manufacturers closely guarded their FEC because it provided a critical competitive advantage. Therefore, coherent transceivers from different vendors could not operate with each other, and a single vendor had to be used for the entire network deployment. However, vendors had to adapt with data center providers pushing disaggregation deeper into communication networks. Their coherent transceivers needed to become interoperable, so FEC algorithms needed standardization. The OIF 400ZR standard for data center interconnects uses a public algorithm called concatenated FEC (CFEC). In contrast, some 400ZR+ MSA standards use open FEC (oFEC), which provides a more extended reach at the cost of a bit more bandwidth and energy consumption. For the longest possible link lengths (500+ kilometers), proprietary FECs become necessary for 400G transmission. Still, at least the public FEC standards have achieved interoperability for a large segment of the 400G transceiver market.
A Smart DSP to Rule All Network Links
A smart pluggable transceiver that can adapt to all the applications we have mentioned before—data centers, carrier networks, SDNs—requires an equally smart and versatile DSP. It must be a DSP that can be reconfigured via software to adapt to different network conditions and use cases. For example, a smart DSP could switch among different FEC algorithms to adapt to network performance and use cases. For example, let’s look at the case of upgrading a long metro link of 650km running at 100 Gbps with open FEC. The operator needs to increase that link capacity to 400 Gbps, but open FEC could struggle to provide the necessary link performance. However, if the DSP can be reconfigured to use a proprietary FEC standard, the transceiver will be able to handle this upgraded link.
400ZR | Open ZR+ | Proprietary Long Haul | |
Target Application | Edge data center interconnect | Metro, Regional data center interconnect | Long-Haul Carrier |
Target Reach @ 400G | 120km | 500km | 1000 km |
Form Factor | QSFP-DD/OSFP | QSFP-DD/OSFP | QSFP-DD/OSFP |
FEC | CFEC | oFEC | Proprietary |
Standards / MSA | OIF | OpenZR+ MSA | Proprietary |
Reconfigurable DSPs can also be beneficial to auto-configure links to deal with specific network conditions, especially in brownfield links. For example, the DSP can be reconfigured to transmit at a higher baud rate if the link has a good quality fiber. However, the DSP could be reconfigured to scale down the baud rate to avoid bit errors if the fiber quality is poor. If the smart pluggable detects that the fiber length is relatively short, it could scale down the laser transmitter power or the DSP power consumption to save energy.
Takeaways
A versatile pluggable that can handle different use cases – data center links, long metro links, and dynamic management and orchestration layers – must have the ability to use different coding and error coding schemes and adapt to different network requirements. The DSP must be equally versatile and switch among several operating modes – 400ZR, 400ZR+, proprietary – and error correction methods – cFEC, oFEC, and proprietary. Together with a programmable optical system on chip, the DSP can not just add software corrections but also make optical hardware changes (output power, turn amplifiers on/off) to adapt to different noise scenarios. Through these adjustments, the next generation of pluggable transceivers will be able to handle all the telecom carrier and data center use cases we can throw at it.