What’s Inside a Tunable Laser for Coherent Systems?
The world is moving towards tunability. The combination of tunable lasers and dense wavelength division multiplexing (DWDM) allows the datacom and telecom industries to expand their network capacity without increasing their existing fiber infrastructure. Furthermore, the miniaturization of coherent technology into pluggable transceiver modules has enabled the widespread implementation of IP over DWDM solutions. Self-tuning algorithms have also made DWDM solutions more widespread by simplifying installation and maintenance. Hence, many application cases—metro transport, data center interconnects, and —are moving towards tunable pluggables.
The tunable laser is a core component of all these tunable communication systems, both direct detection and coherent. The laser generates the optical signal modulated and sent over the optical fiber. Thus, the purity and strength of this signal will have a massive impact on the bandwidth and reach of the communication system. This article will clarify some critical aspects of laser design for communication systems.
External and Integrated Lasers: What’s the Difference?
The promise of silicon photonics (SiP) is compatibility with existing electronic manufacturing ecosystems and infrastructure. Integrating silicon components on a single chip with electronics manufacturing processes can dramatically reduce the footprint and the cost of optical systems and open avenues for closer integration with silicon electronics on the same chip. However, the one thing silicon photonics misses is the laser component.
Silicon is not a material that can naturally emit laser light from electrical signals. Decades of research have created silicon-based lasers with more unconventional nonlinear optical techniques. Still, they cannot match the power, efficiency, tunability, and cost-at-scale of lasers made from indium phosphide (InP) and III-V compound semiconductors.
Therefore, making a suitable laser for silicon photonics does not mean making an on-chip laser from silicon but an external laser from III-V materials such as InP. This light source will be coupled via optical fiber to the silicon components on the chip while maintaining a low enough footprint and cost for high-volume integration. The external laser typically comes in the form of an integrable tunable laser assembly (ITLA).
In contrast, the InP platform can naturally emit light and provide high-quality light sources and amplifiers. This allows for photonic system-on-chip designs that include an integrated laser on the chip. The integrated laser carries the advantage of reduced footprint and power consumption compared to an external laser. These advantages become even more helpful for PICs that need multiple laser channels.
Finally, integrated lasers enable earlier optical testing on the semiconductor wafer and die. By testing the dies and wafers directly before packaging them into a transceiver, manufacturers need only discard the bad dies rather than the whole package, which saves valuable energy, materials, and cost.
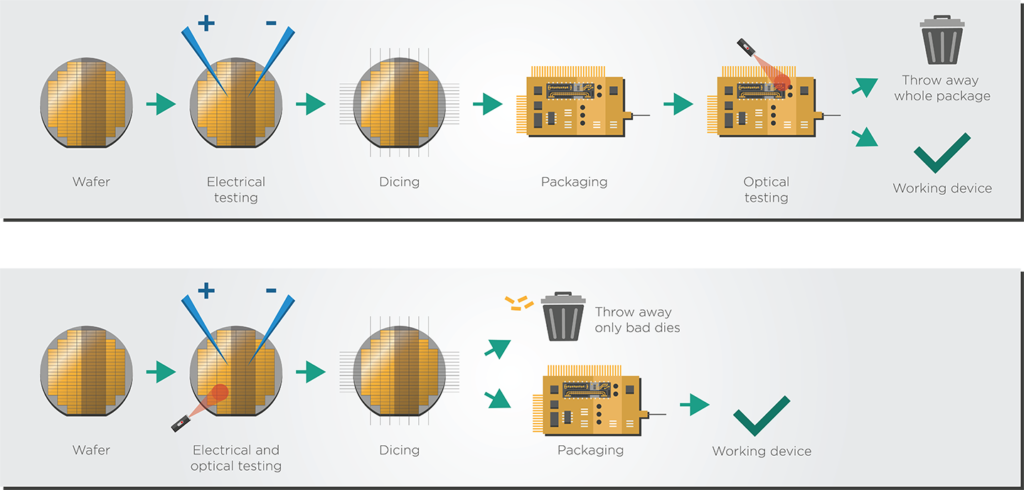
Using an external or integrated laser depends on the transceiver developer’s device requirements, supply chain, and manufacturing facilities and processes. At EFFECT Photonics, we have the facilities and expertise to provide fully-integrated InP optical systems with an integrated laser and the external laser component that a silicon photonics developer might need for their optical system.
What are the key requirements for a laser in coherent systems?
In his recent talk at ECOC 2022, our Director of Product Management, Joost Verberk, outlined five critical parameters for laser performance.
- Tunability: With telecom providers needing to scale up their network capacity without adding more fiber infrastructure, combining tunable lasers with dense wavelength division multiplexing (DWDM) technology becomes necessary. These tunable optical systems have become more widespread thanks to self-tuning technology that removes the need for manual tuning. This makes their deployment and maintenance easier.
- Spectral Purity: Coherent systems encode information in the phase of the light, and the purer the light source is, the more information it can transmit. An ideal, perfectly pure light source can generate a single, exact color of light. However, real-life lasers are not pure and will generate light outside their intended color. The size of this deviation is what we call the laser linewidth. An impure laser with a large linewidth will have a more unstable phase that propagates errors in its transmitted data, as shown in the diagram below. This means it will transmit at a lower speed than desired.
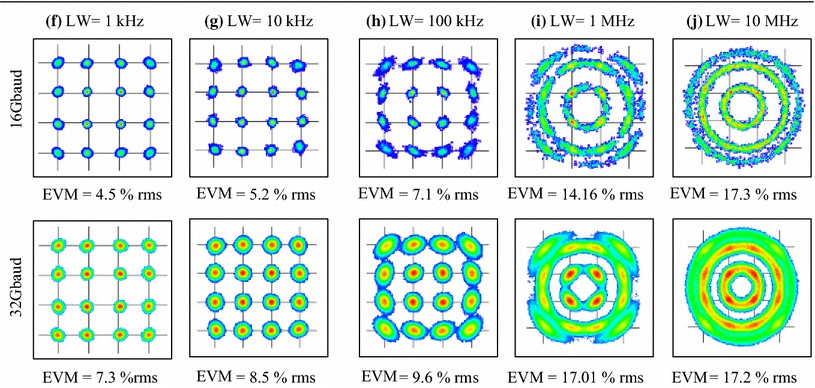
- Dimensions: As the industry moves towards packing more and more transceivers on a single router faceplate, tunable lasers need to maintain performance and power while moving to smaller footprints. Laser manufacturers have achieved size reductions thanks to improved integration without impacting laser purity and power, moving from ITLA to micro-ITLA and nano-ITLA form factors in a decade.
- Environmental Resistance: Lasers used in edge and access networks will be subject to harsh environments, like temperature and moisture changes. For these use cases, lasers should operate in the industrial temperature (I-temp) range of -40 to 85ºC for these environments.
- Transmit Power: The required laser output power will depend on the application and the system architecture. For example, a laser fully integrated into the chip can reach higher transmit powers more easily because it avoids the interconnection losses of an external laser. Still, shorter-reach applications might not necessarily need such powers.
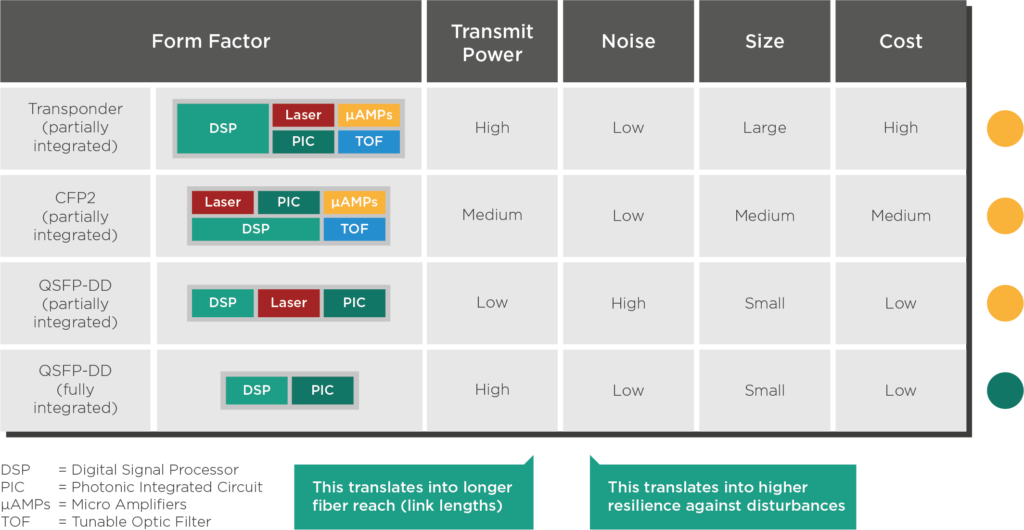
The Promise of Multi-Laser Arrays
Earlier this year, Intel Labs demonstrated an eight-wavelength laser array fully integrated on a silicon wafer. These milestones are essential for tunable DWDM because the laser arrays can allow for multi-channel transceivers that are more cost-effective when scaling up to higher speeds.
Let’s say we need a link with 1.6 Terabits/s of capacity. There are three ways we could implement it:
- Four modules of 400G: This solution uses existing off-the-shelf modules but has the largest footprint. It requires four slots in the router faceplate and an external multiplexer to merge these into a single 1.6T channel.
- One module of 1.6T: This solution will not require the external multiplexer and occupies just one plug slot on the router faceplate. However, making a single-channel 1.6T device has the highest complexity and cost.
- One module with four internal channels of 400G: A module with an array of four lasers (and thus four different 400G channels) will only require one plug slot on the faceplate while avoiding the complexity and cost of the single-channel 1.6T approach.
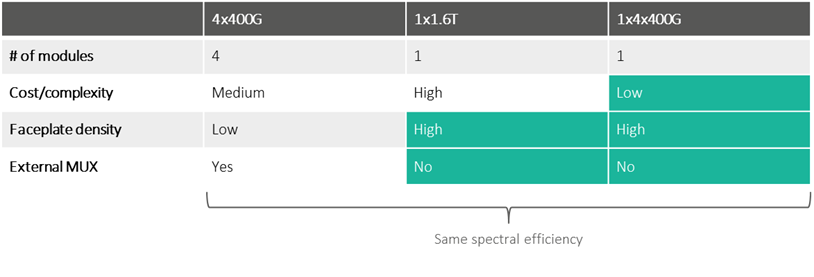
Multi-laser array and multi-channel solutions will become increasingly necessary to increase link capacity in coherent systems. They will not need more slots in the router faceplate while simultaneously avoiding the higher cost and complexity of increasing the speed with just a single channel.
Takeaways
The combination of tunable lasers and dense wavelength division multiplexing (DWDM) allows the datacom and telecom industries to expand their network capacity without increasing their existing fiber infrastructure. Thanks to the miniaturization of coherent technology and self-tuning algorithms, many application cases—metro transport, data center interconnects, and future access networks—will eventually move towards coherent tunable pluggables.
These new application cases will have to balance the laser parameters we described early—tunability, purity, size, environmental resistance, power—depending on their material platforms, system architecture, and requirements. Some will need external lasers; some will want a fully-integrated laser. Some will need multi-laser arrays to increase capacity; others need more stringent temperature certifications.
Following these trends, at EFFECT Photonics, we are not only developing the capabilities to provide a complete, coherent transceiver solution but also the external nano-ITLA units needed by other vendors.