What is Laser Linewidth and Why Does it Matter
The world is moving towards tunability. The combination of tunable lasers and dense wavelength division multiplexing (DWDM) allows the datacom and telecom industries to expand their network capacity without increasing their existing fiber infrastructure. Furthermore, the miniaturization of coherent technology into pluggable transceiver modules has enabled the widespread implementation of IP over DWDM solutions. Self-tuning algorithms have also made DWDM solutions more widespread by simplifying installation and maintenance. Hence, many application cases—metro transport, data center interconnects, and —are moving towards tunable pluggables.
The tunable laser is a core component of all these tunable communication systems, both direct detection and coherent. The laser generates the optical signal modulated and sent over the optical fiber. Thus, the purity and strength of this signal will have a massive impact on the bandwidth and reach of the communication system.
What is laser linewidth?
Coherent systems encode information in the phase of the light, and the purer the light source is, the more information it can transmit. An ideal, perfectly pure light source can generate a single, exact color of light. However, real-life lasers are not pure and will generate light outside their intended color. The size of this deviation is what we call the laser linewidth. In other words, the linewidth describes the range of wavelengths present in the wavelength spectrum of the laser beam.
The linewidth of a laser can be defined in different ways depending on the specific criteria used. Here are a few examples:
- Full Width at Half Maximum (FWHM): This is a common and straightforward definition. It refers to the width of the laser spectrum at the point where the intensity is half its maximum.
- Gaussian Linewidth: In some cases, the linewidth can be characterized by the standard deviation of a Gaussian distribution that fits the spectral profile of the laser output.
- Schawlow-Townes Linewidth: This definition is associated with the quantum noise of the laser. You could consider this the fundamental, smallest possible linewidth an “ideal” laser could have.
- Lorentzian Linewidth: The Lorentzian linewidth is based on the Lorentzian distribution, often used to model the spectral lines of certain lasers.
- Frequency or Wavelength Range: Instead of using a specific criterion like FWHM, some applications may define linewidth by specifying the frequency or wavelength range within which a certain percentage (e.g., 95%) of the total power is contained.
These different definitions may be more suitable for specific contexts or applications, depending on the requirements and characteristics of the laser system in question.
What Impact Does Linewidth Have on Coherent Transmission?
A laser is a very precise generator of light signals. Phase noise is like a tiny, random wobble or instability in the timing of these signals. It’s as if the laser can’t decide exactly when to start and stop its light output, creating a small amount of uncertainty in the timing. Precise timing is everything for communication applications.
An impure laser with a large linewidth will have a more unstable phase that propagates errors in its transmitted data, as shown in the diagram below. This means it will transmit at a lower speed than desired.
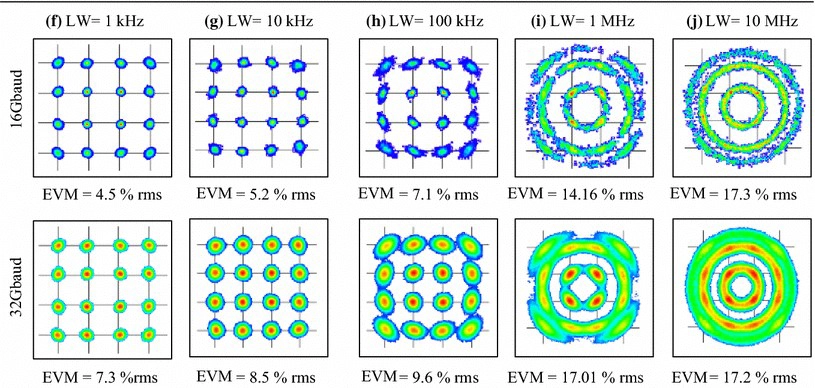
What are Some Ways to Reduce Laser Linewidth and Noise?
One of the most straightforward ways to improve the linewidth of a semiconductor laser is to use it inside a second, somewhat larger resonator. This setup is called an external cavity laser (ECL) since this new resonator or cavity will use additional optical elements external to the original laser.
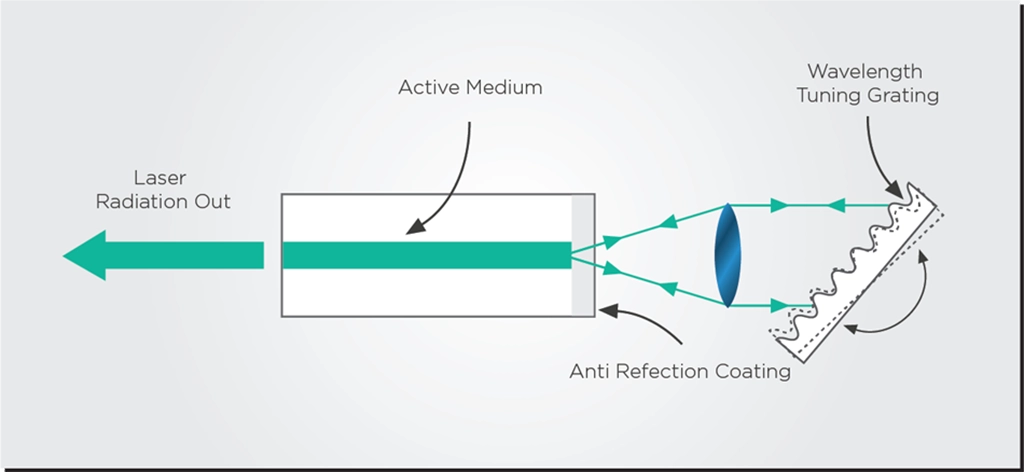
The new external resonator also provides more degrees of freedom for tuning the laser. ECLs have become the state-of-the-art solution in the telecom industry: they use a DFB or DBR laser as the “base laser” and external gratings as their filtering element for additional tuning. These lasers can provide a high-quality laser beam with low noise, narrow linewidth, and a wide tuning range. However, they came with a cost: manufacturing complexity.
EFFECT Photonics takes a very different approach to building lasers. Most developers make their lasers using linear resonators in which the laser light bounces back and forth between two mirrors. However, EFFECT Photonics uses ring resonators, which take a different approach to feedback: the light loops multiple times inside a ring that contains the active medium. The ring is coupled to the rest of the optical circuit via a waveguide.
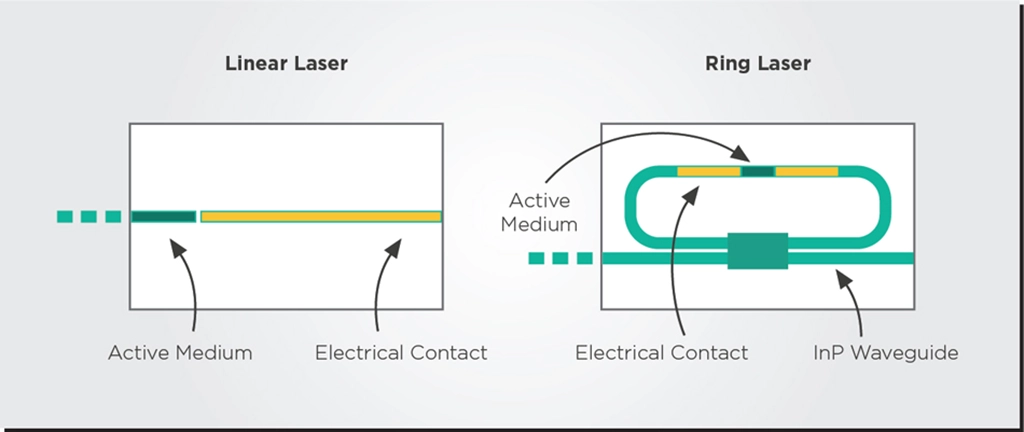
The power of the ring resonator lies in its compactness, flexibility, and integrability. While a single ring resonator is not that impressive or tunable, using multiple rings and other optical elements allows them to achieve linewidth and tunability on par with the state-of-the-art tunable lasers that use linear resonators.
Takeaways
Laser linewidth, which describes the range of wavelengths in the laser beam, is paramount in coherent optical transmission systems. In such systems, where information is encoded in the phase of light, a purer light source allows for transmitting more information. A narrower laser linewidth corresponds to a more stable phase, reducing phase noise and enhancing the signal quality.
Techniques such as external cavity lasers (ECL) have been employed to improve linewidth, offering a high-quality laser beam with low noise and narrow linewidth. Alternatively, EFFECT Photonics employs ring resonators, providing an innovative approach to achieving linewidth and tunability comparable to state-of-the-art tunable lasers while emphasizing compactness and integrability.