The Power of Monolithic Lasers
Over the last decade, technological progress in tunable laser integration has matched the need for smaller footprints. In 2011, tunable lasers followed the multi-source agreement (MSA) for integrable tunable laser assemblies (ITLAs). By 2015, tunable lasers were sold in the more compact micro-ITLA form factor, constituting a mere 22% of the original ITLA package volume. In 2019, the nano-ITLA form factor reduced ITLA volumes further, as the module was just 39% of the micro-ITLA volume.
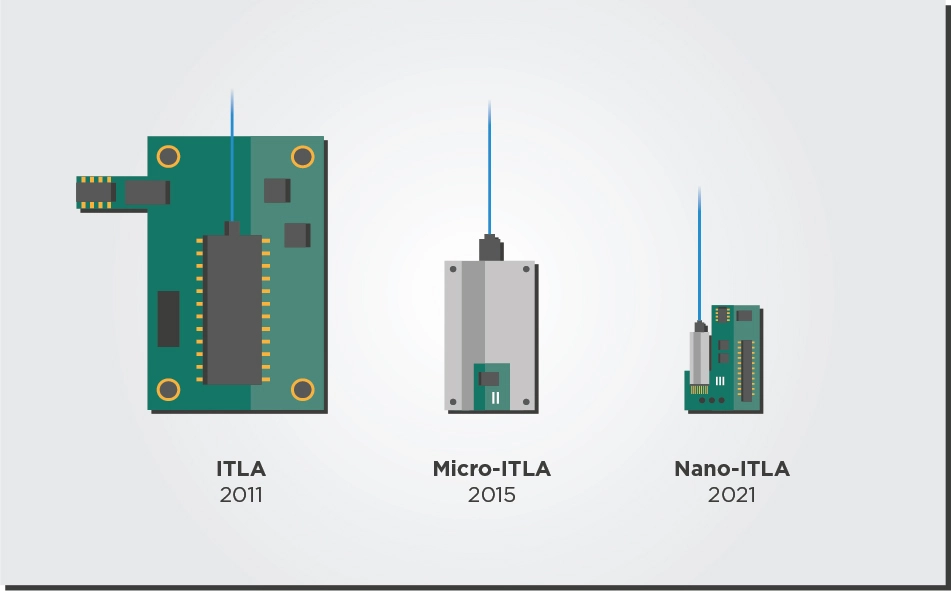
Despite this progress, the industry will need further laser integration for the QSFP28 pluggables used in 100G ZR coherent access. Since QSFP28 pluggables have a lower power consumption and slightly smaller footprint than QSFP-DD modules, they should not use the same lasers as in QSFP-DD modules. They need specialized laser solutions with a smaller footprint and lower power consumption.
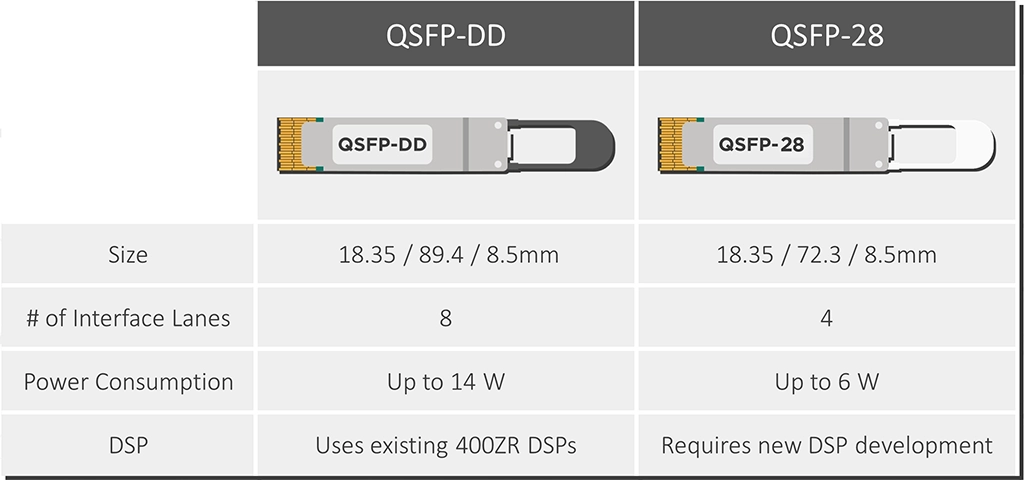
Achieving these ambitious targets requires monolithic lasers that ideally include all key laser functions (gain, laser cavity, and wavelength locker) on the same chip. In this article, we will review the work from EFFECT Photonics and other laser developers to reach this monolithic integration goal.
External Cavity Lasers Are the Industry Standard
One of the most straightforward ways to improve the quality and tunability of a semiconductor laser is to use it inside a second, somewhat larger resonator. This setup is called an external cavity laser (ECL) since this new resonator or cavity will use additional optical elements external to the original laser.
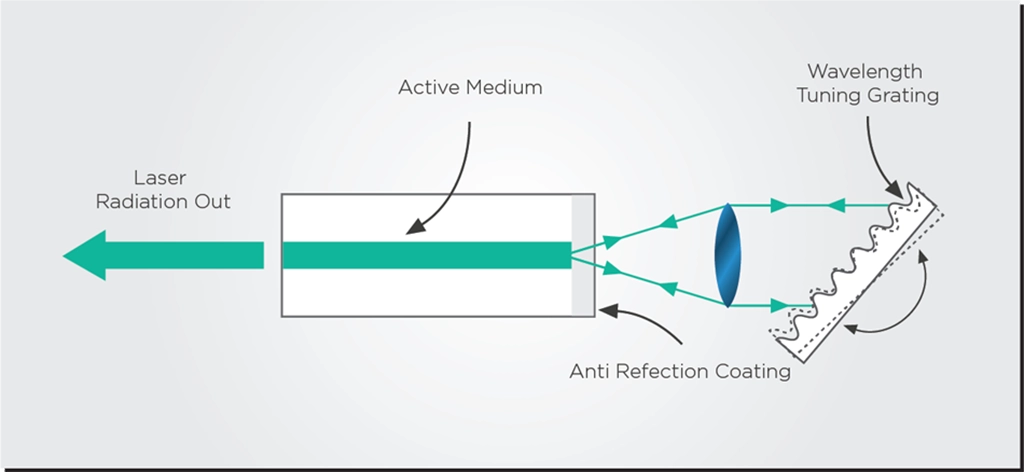
The new external resonator provides more degrees of freedom for tuning the laser. If the resonator uses a mirror, then the laser can be tuned by moving the mirror a bit and changing the length of the resonator. If the resonator uses a grating, it has an additional element to tune the laser by filtering.
ECLs have become the state-of-the-art solution in the telecom industry: they use a DFB or DBR laser as the “base laser” and external gratings as their filtering element for additional tuning. These lasers can provide a high-quality laser beam with low noise, narrow linewidth, and a wide tuning range. However, they came with a cost: manufacturing complexity.
ECLs initially required free-space bulk optical elements, such as lenses and mirrors, for the external cavity. One of the hardest things to do in photonics is coupling between free-space optics and a chip. This alignment of the free-space external cavity with the original laser chip is extremely sensitive to environmental disturbances. Therefore, their coupling is often inefficient and complicates manufacturing and assembly processes, making these lasers much harder to manufacture in volume.
Re-Integrating the Laser Cavity
In 2019, researchers from NeoPhotonics (now part of Lumentum) published a laser design that tried to overcome this obstacle by manufacturing the external cavity on a separate chip coupled to the original laser chip. Coupling these two chips together still entails some manufacturing complexity, but it is a more feasible and scalable setup than coupling from chip to free space optics.
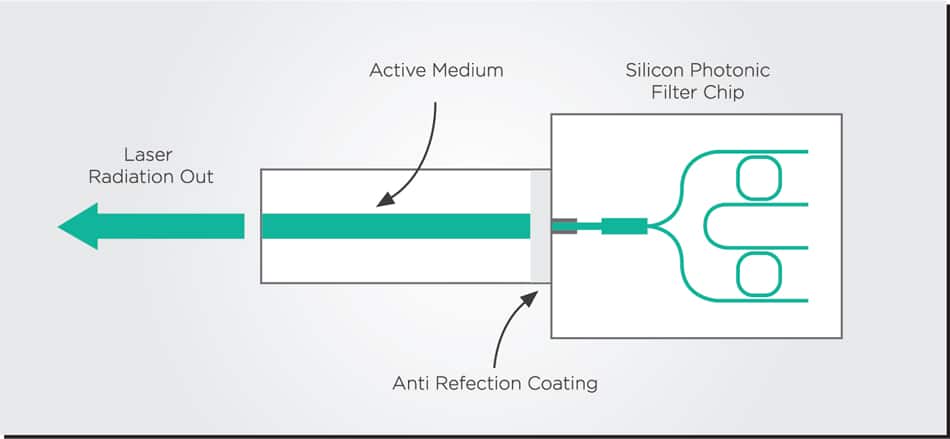
In 2021, Furukawa announced a new laser design for their upcoming nano-ITLA. This design fully reintegrates the laser cavity on an InP gain chip while still meeting the power and tunability required of the laser. This was tremendous progress, but one component remained elusive to integrate on the same chip: the wavelength locker.
Every tunable laser needs a wavelength locker component that can stabilize the laser’s output regardless of environmental conditions such as temperature. This component is usually an additional optical resonator that requires coupling from chip to free space or from chip to glass. In the case of the Furukawa design, a small wavelength locker was made in glass, which made the coupling to the chip slightly easier but still lossy.
Full Monolithic Integration with the Wavelength Locker
EFFECT Photonics takes a very different approach to building lasers. Most developers make their lasers using linear resonators in which the laser light bounces back and forth between two mirrors. However, EFFECT Photonics uses ring resonators, which take a different approach to feedback: the light loops multiple times inside a ring that contains the active medium. The ring is coupled to the rest of the optical circuit via a waveguide.
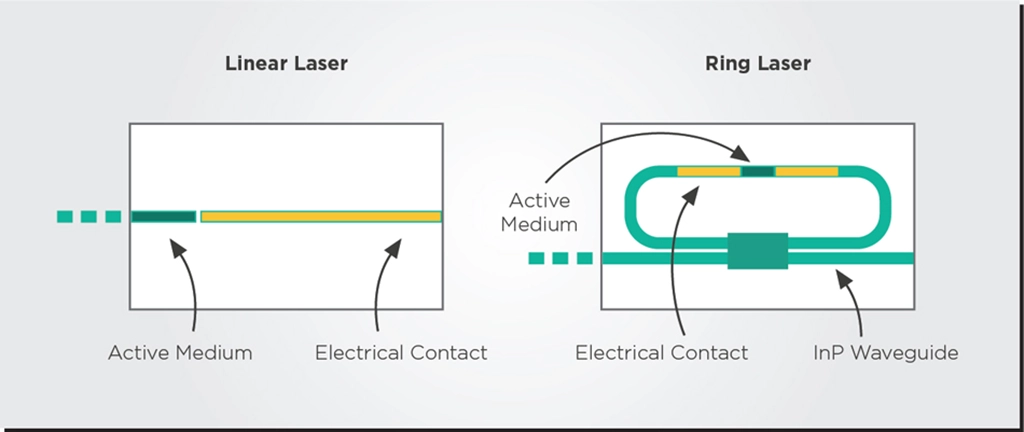
The power of the ring resonator lies in its compactness, flexibility, and integrability. While a single ring resonator is not that impressive or tunable, using multiple rings and other optical elements allows them to achieve performance and tunability on par with the state-of-the-art tunable lasers that use linear resonators.
Most importantly, these widely tunable ring lasers can be entirely constructed on a single chip of Indium Phosphide (InP) material. This enables the development of a tunable laser whose functions are all monolithically integrated on a single InP chip. This includes the gain section, laser cavity, optical amplifier, and wavelength locker onto one chip.
This monolithic integration of all tunable laser functions allows EFFECT Photonics to develop a novel pico-ITLA (pITLA) module that will become the world’s smallest ITLA for coherent applications. The pITLA is the next step in tunable laser integration, including all laser functions in a package with just 20% of the volume of a nano-ITLA module. The figure below shows that even a standard matchstick dwarves the pITLA in size.
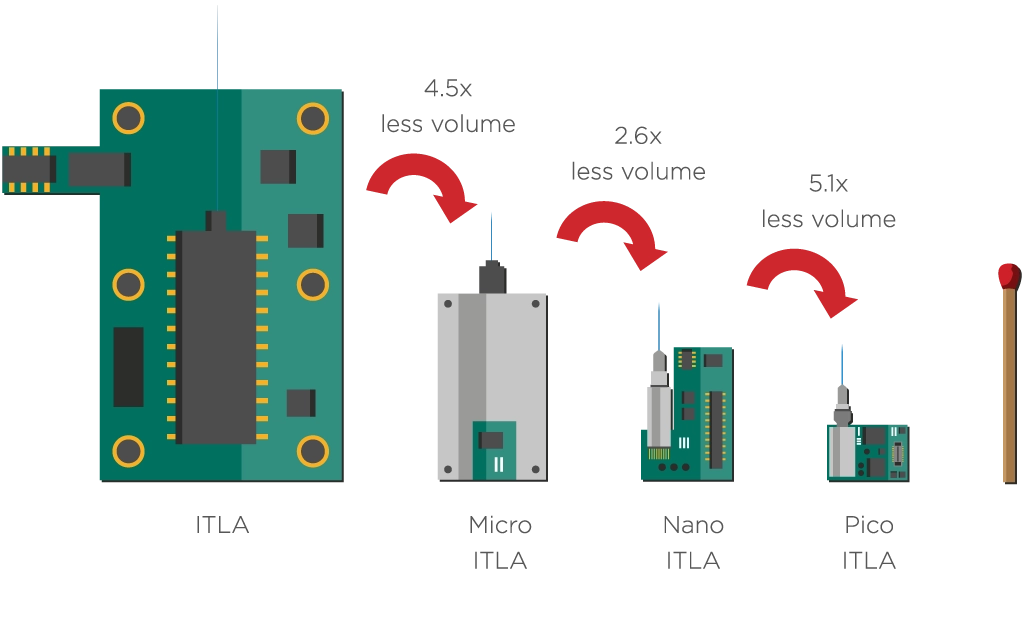
Takeaways
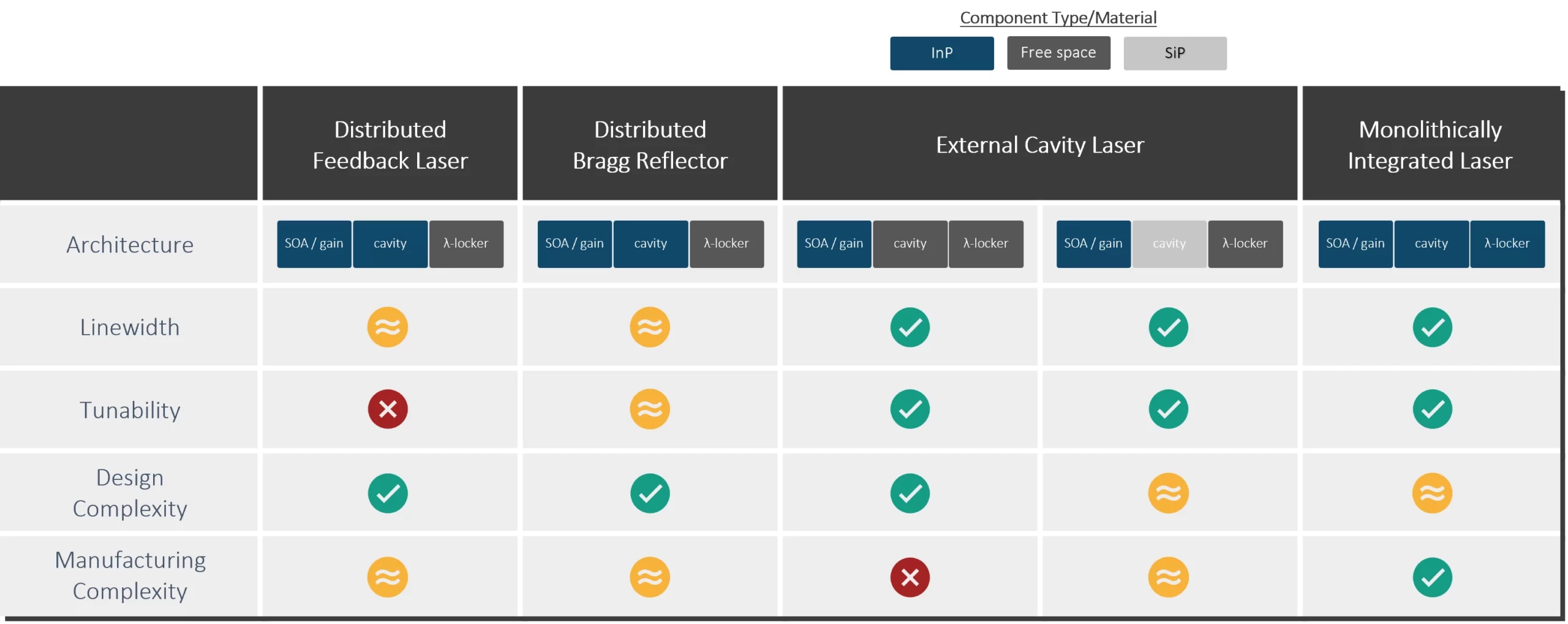
External cavity lasers have been the industry telecom standards, but they are difficult to manufacture in high volumes. Ideally, the best way to scale laser production is to integrate as many lasers functions as possible (gain medium, laser cavity, and wavelength locker) on the same chip. The laser can then be manufactured with a high-volume semiconductor process.
EFFECT Photonics’ laser solution is unique because it enables a widely tunable and monolithically integrated laser with all elements, including wavelength locker, on the same chip. This setup is ideal for scaling into high production volumes.