The Future of Passive Optical Networks
Like every other telecom network, cable networks had to change to meet the growing demand for data. These demands led to the development of hybrid fiber-coaxial (HFC) networks in the 1990s and 2000s. In these networks, optical fibers travel from the cable company hub and terminate in optical nodes, while coaxial cable connects the last few hundred meters from the optical node to nearby houses. Most of these connections were asymmetrical, giving customers more capacity to download data than upload.
That being said, the way we use the Internet has evolved over the last ten years. Users now require more upstream bandwidth thanks to the growth of social media, online gaming, video calls, and independent content creation such as video blogging. The DOCSIS standards that govern data transmission over coaxial cables have advanced quickly because of these additional needs. For instance, full-duplex transmission with symmetrical upstream and downstream channels is permitted under the most current DOCSIS 4.0 specifications.
Fiber-to-the-home (FTTH) systems, which bring fiber right to the customer’s door, are also proliferating and enabling Gigabit connections quicker than HFC networks. Overall, extending optical fiber deeper into communities (see Figure 1 for a graphic example) is a critical economic driver, increasing connectivity for the rural and underserved. These investments also lead to more robust competition among cable companies and a denser, higher-performance wireless network.

Passive optical networks (PONs) are a vital technology to cost-effectively expand the use of optical fiber within access networks and make FTTH systems more viable. By creating networks using passive optical splitters, PONs avoid the power consumption and cost of active components in optical networks such as electronics and amplifiers. PONs can be deployed in mobile fronthaul and mid-haul for macro sites, metro networks, and enterprise scenarios.
Despite some success from PONs, the cost of laying more fiber and the optical modems for the end users continue to deter carriers from using FTTH more broadly across their networks. This cost problem will only grow as the industry moves into higher bandwidths, such as 50G and 100G, requiring coherent technology in the modems.
Therefore, new technology and manufacturing methods are required to make PON technology more affordable and accessible. For example, wavelength division multiplexing (WDM)-PON allows providers to make the most of their existing fiber infrastructure. Meanwhile, simplified designs for coherent digital signal processors (DSPs) manufactured at large volumes can help lower the cost of coherent PON technology for access networks.
The Advantages of WDM PONs
Previous PON solutions, such as Gigabit PON (GPON) and Ethernet PON (EPON), used time-division multiplexing (TDM) solutions. In these cases, the fiber was shared sequentially by multiple channels. These technologies were initially meant for the residential services market, but they scale poorly for the higher capacity of business or carrier services. PON standardization for 25G and 50G capacities is ready but sharing a limited bitrate among multiple users with TDM technology is an insufficient approach for future-proof access networks.
This WDM-PON uses WDM multiplexing/demultiplexing technology to ensure that data signals can be divided into individual outgoing signals connected to buildings or homes. This hardware-based traffic separation gives customers the benefits of a secure and scalable point-to-point wavelength link. Since many wavelength channels are inside a single fiber, the carrier can retain very low fiber counts, yielding lower operating costs.
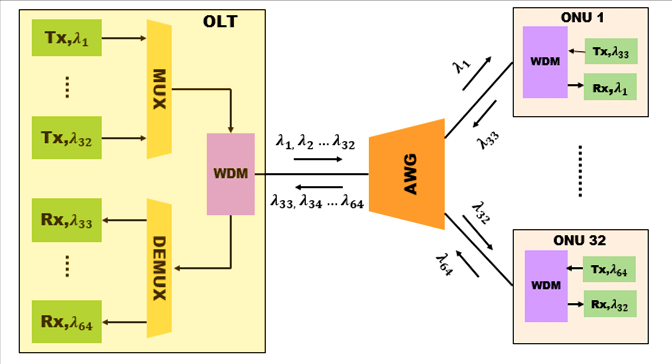
WDM-PON has the potential to become the unified access and backhaul technology of the future, carrying data from residential, business, and carrier wholesale services on a single platform. We discussed this converged access solution in one of our previous articles. Its long-reach capability and bandwidth scalability enable carriers to serve more customers from fewer active sites without compromising security and availability.
Migration to the WDM-PON access network does require a carrier to reassess how it views its network topology. It is not only a move away from operating parallel purpose-built platforms for different user groups to one converged access and backhaul infrastructure. It is also a change from today’s power-hungry and labor-intensive switch and router systems to a simplified, energy-efficient, and transport-centric environment with more passive optical components.
The Possibility of Coherent Access
As data demands continue to grow, direct detect optical technology used in prior PON standards will not be enough. The roadmap for this update remains a bit blurry, with different carriers taking different paths. For example, future expansions might require using 25G or 50G transceivers in the cable network, but the required number of channels in the fiber might not be enough for the conventional optical band (the C-band). Such a capacity expansion would therefore require using other bands (such as the O-band), which comes with additional challenges. An expansion to other optical bands would require changes in other optical networking equipment, such as multiplexers and filters, which increases the cost of the upgrade.
An alternative solution could be upgrading instead to coherent 100G technology. An upgrade to 100G could provide the necessary capacity in cable networks while remaining in the C-band and avoiding using other optical bands. This path has also been facilitated by the decreasing costs of coherent transceivers, which are becoming more integrated, sustainable, and affordable. You can read more about this subject in one of our previous articles.
For example, the renowned non-profit R&D center CableLabs announced a project to develop a symmetric 100G Coherent PON (C-PON). According to CableLabs, the scenarios for a C-PON are many: aggregation of 10G PON and DOCSIS 4.0 links, transport for macro-cell sites in some 5G network configurations, fiber-to-the-building (FTTB), long-reach rural scenarios, and high-density urban networks.
CableLabs anticipates C-PON and its 100G capabilities to play a significant role in the future of access networks, starting with data aggregation on networks that implement a distributed access architecture (DAA) like Remote PH. You can learn more about these networks here.
Combining Affordable Designs with Affordable Manufacturing
The main challenge of C-PON is the higher cost of coherent modulation and detection. Coherent technology requires more complex and expensive optics and digital signal processors (DSPs). Plenty of research is happening on simplifying these coherent designs for access networks. However, a first step towards making these optics more accessible is the 100ZR standard.
100ZR is currently a term for a short-reach (~80 km) coherent 100Gbps transceiver in a QSFP pluggable size. Targeted at the metro edge and enterprise applications that do not require 400ZR solutions, 100ZR provides a lower-cost, lower-power pluggable that also benefits from compatibility with the large installed base of 50 GHz and legacy 100 GHz multiplexer systems.
Another way to reduce the cost of PON technology is through the economics of scale, manufacturing pluggable transceiver devices at a high volume to drive down the cost per device. And with greater photonic integration, even more, devices can be produced on a single wafer. This economy-of-scale principle is the same behind electronics manufacturing, which must be applied to photonics.
Researchers at the Technical University of Eindhoven and the JePPIX consortium have modeled how this economy of scale principle would apply to photonics. If production volumes can increase from a few thousand chips per year to a few million, the price per optical chip can decrease from thousands of Euros to tens of Euros. This must be the goal of the optical transceiver industry.
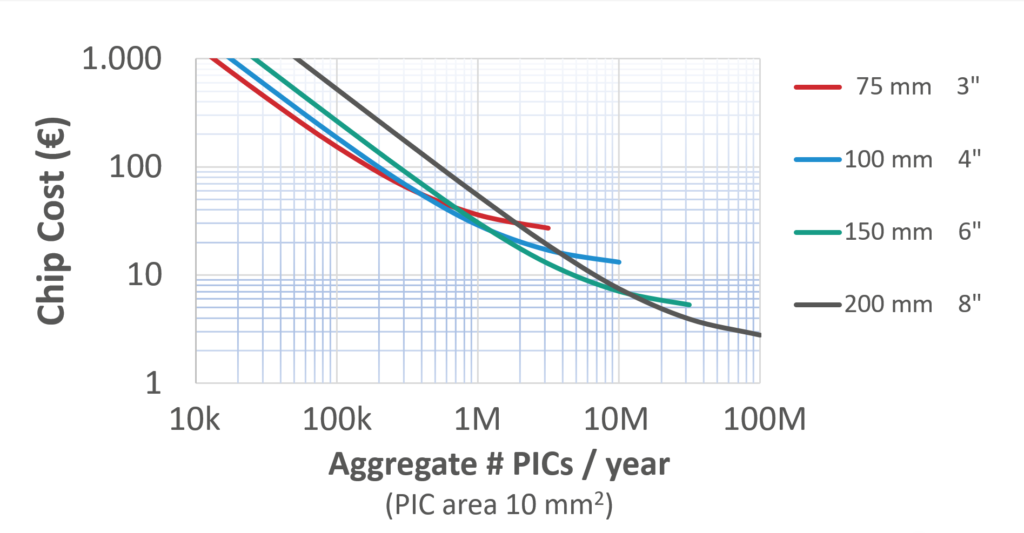
Takeaways
Integrated photonics and volume manufacturing will be vital for developing future passive optical networks. PONs will use more WDM-PON solutions for increased capacity, secure channels, and easier management through self-tuning algorithms.
Meanwhile, PONs are also moving into incorporating coherent technology. These coherent transceivers have been traditionally too expensive for end-user modems. Fortunately, more affordable coherent transceiver designs and standards manufactured at larger volumes can change this situation and decrease the cost per device.