What are FEC and PCS, and Why do They Matter?
Coherent transmission has become a fundamental component of optical networks to address situations where direct detect technology cannot provide the required capacity and reach.
While Direct Detect transmission only uses the amplitude of the light signal, Coherent optical transmission manipulates three different light properties: amplitude, phase, and polarization. These additional degrees of modulation allow for faster optical signals without compromising the transmission distance. Furthermore, coherent technology enables capacity upgrades without replacing the expensive physical fiber infrastructure on the ground.
However, the demand for data never ceases, and with it, developers of digital signal processors (DSPs) have had to figure out ways to improve the efficiency of coherent transmission. In this article, we will briefly describe the impact of two algorithms that DSP developers use to make coherent transmission more efficient: Forward Error Correction (FEC) and Probabilistic Constellation Shaping (PCS).
What is Forward Error Correction?
Forward Error Correction (FEC) implemented by DSPs has become a vital component of coherent communication systems. FEC makes the coherent link much more tolerant to noise than a direct detect system and enables much longer reach and higher capacity. Thanks to FEC, coherent links can handle bit error rates that are literally a million times higher than a typical direct detect link.
Let’s provide a high-level overview of how FEC works. An FEC encoder adds a series of redundant bits (called overhead) to the transmitted data stream. The receiver can use this overhead to check for errors without asking the transmitter to resend the data.
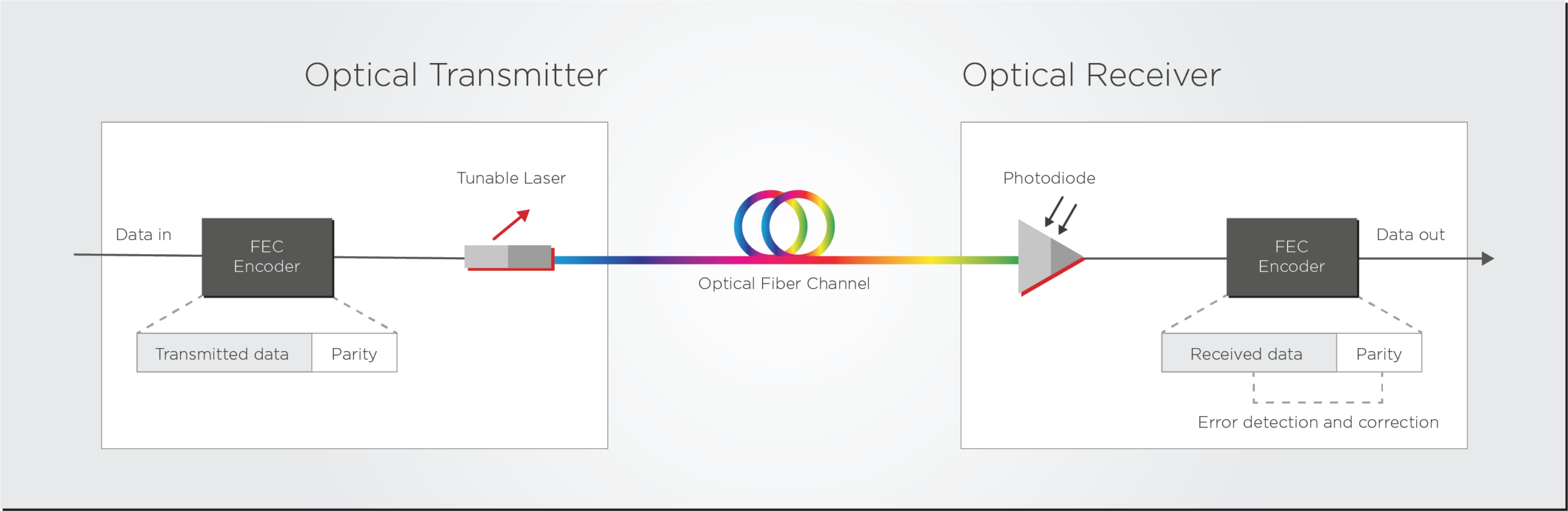
In other words, FEC algorithms allow the DSP to enhance the link performance without changing the hardware. This enhancement is analogous to imaging cameras: image processing algorithms allow the lenses inside your phone camera to produce a higher-quality image.
We must highlight that FEC is a block of an electronic DSP engine with its own specialized circuitry and algorithms, so it is a separate piece of intellectual property. Therefore, developing the entire DSP electronic engine (see Figure 2 for the critical component blocks of a DSP) requires ownership or access to specific FEC intellectual property.
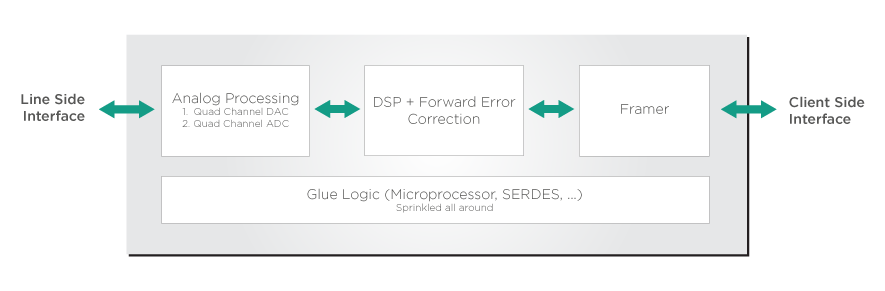
What is Probabilistic Constellation Shaping?
DSP developers can transmit more data by transmitting more states in their quadrature-amplitude modulation process. The simplest kind of QAM (4-QAM) uses four different states (usually called constellation points), combining two different intensity levels and two different phases of light.
By using more intensity levels and phases, more bits can be transmitted in one go. State-of-the-art commercially available 400ZR transceivers typically use 16-QAM, with sixteen different constellation points that arise from combining four different intensity levels and four phases. However, this increased transmission capacity comes at a price: a signal with more modulation orders is more susceptible to noise and distortions. That’s why these transceivers can transmit 400Gbps over 100km but not over 1000km.
One of the most remarkable recent advances in DSPs to increase the reach of light signals is Probabilistic Constellation Shaping (PCS). In the typical 16-QAM modulation used in coherent transceivers, each constellation point has the same probability of being used. This is inefficient since the outer constellation points that require more power have the same probability as the inner constellation points that require lower power.
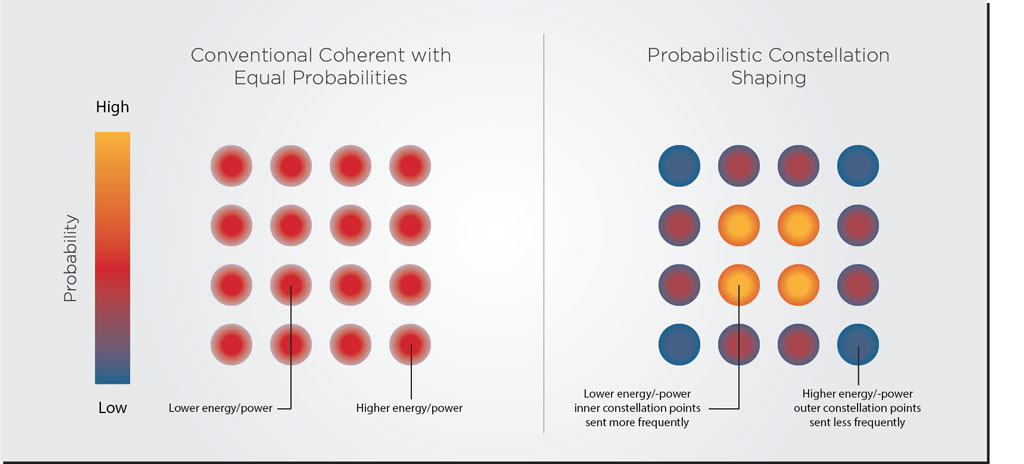
PCS uses the low-power inner constellation points more frequently and the outer constellation points less frequently, as shown in Figure 3. This feature provides many benefits, including improved tolerance to distortions and easier system optimization to specific bit transmission requirements. If you want to know more about it, please read the explainers here and here.
The Importance of Standardization and Reconfigurability
Algorithms like FEC and PCS have usually been proprietary technologies. Equipment and component manufacturers closely guarded their algorithms because they provided a critical competitive advantage. However, this often meant that coherent transceivers from different vendors could not operate with each other, and a single vendor had to be used for the entire network deployment.
Over time, coherent transceivers have increasingly needed to become interoperable, leading to some standardization in these algorithms. For example, the 400ZR standard for data center interconnects uses a public algorithm called concatenated FEC (CFEC). In contrast, some 400ZR+ MSA standards use open FEC (oFEC), which provides a more extended reach at the cost of a bit more bandwidth and energy consumption. For the longest possible link lengths (500+ kilometers), proprietary FECs become necessary for 400G transmission. Still, at least the public FEC standards have achieved interoperability for a large segment of the 400G transceiver market. Perhaps in the future, this could happen with PCS methods.
Future DSPs could switch among different algorithms and methods to adapt to network performance and use cases. For example, let’s look at the case of upgrading a long metro link of 650km running at 100 Gbps with open FEC. The operator needs to increase that link capacity to 400 Gbps, but open FEC could struggle to provide the necessary link performance. However, if the DSP can be reconfigured to use a proprietary FEC standard, the transceiver will be able to handle this upgraded link. Similarly, longer reach could be achieved if the DSP activates its PCS feature.
400 ZR | Open ZR+ | Proprietary Long Haul | |
Target Application | Edge data center interconnect | Metro, Regional data center interconnect | Long-Haul Carrier |
Target Reach @ 400G | 120km | 500km | 1000 km |
Form Factor | QSFP-DD/OSFP | QSFP-DD/OSFP | QSFP-DD/OSFP |
FEC | CFEC | oFEC | Proprietary |
Standards / MSA | OIF | OpenZR+ MSA | Proprietary |
Takeaways
The entire field of communication technology can arguably be summarized with a single question: how can we transmit more information into a single frequency-limited signal over the longest possible distance?
DSP developers have many tools to answer this question, and two of them are FEC and PCS. Both technologies make coherent links much more tolerant of noise and can extend their reach. Future pluggables that handle different use cases must use different coding, error coding, and modulation schemes to adapt to different network requirements.
There are still many challenges ahead to improve DSPs and make them transmit even more bits in more energy-efficient ways. Now that EFFECT Photonics has incorporated talent and intellectual property from Viasat’s Coherent DSP team, we hope to contribute to this ongoing research and development and make transceivers faster and more sustainable than ever.