The Highways of Light: How Optical Fiber Works
Optical fibers revolutionized how we transmit data, enabling faster long-distance connections. These slender strands of glass or plastic carry light pulses and serve as the backbone of modern telecommunication networks. Optical fibers have found applications beyond communications, including imaging, sensing, and medicine, further showcasing their versatility and impact in various fields.
Early optical fibers suffered significant losses during transmission, limiting their practicality for long-haul communication. In 1966, Charles Kao and George Hockham proposed that impurities in the glass were responsible for these losses, and they suggested that high-purity silica glass could achieve a much more reasonable attenuation of 20 dB per kilometer for telecommunications. This breakthrough, for which Kao received the Nobel Prize in Physics in 2009, kickstarted an era of explosive progress and growth for optical fiber.
In 1970, Corning scientists Robert Maurer, Donald Keck, and Peter Schultz successfully fabricated a glass fiber with an attenuation of 16 dB per kilometer, exceeding the performance benchmark set by Kao and Hockham. Two years later, Corning pushed the envelope further and achieved a loss of 4 dB/km, an order of magnitude improvement over their first effort. By 1979, Nippon Telegraph and Telephone (NTT) had reached a loss of 0.2 dB/km, meaning that only 5% of the light signal was lost over one kilometer. Optical fibers were ready for the world stage and deployed worldwide throughout the 1980s. The first transatlantic optical fiber link, spanning 6000 km, was established in 1988.
In this article, we will delve into the fascinating world of optical fibers, exploring how they work and what role optical transceivers play in fiber communications.
The Principle of Total Internal Reflection
Light bends when it passes from one material to another, such as from air to water. This bending occurs due to the change in the speed of light when it encounters a different material, causing the light rays to change direction. How much light changes direction depends on the angle at which it enters the new material and the factor by which the material slows down light. The latter factor is known as the refractive index of the material.
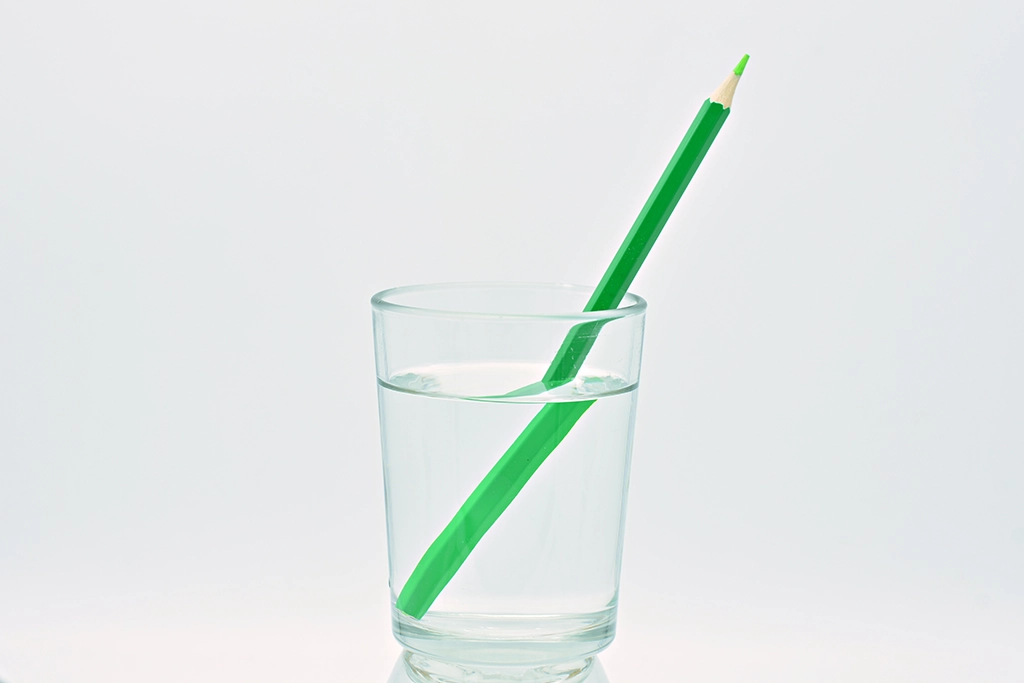
When light travels from a material of a higher refractive index to one of a lower index at a specific critical angle, the light will be entirely reflected into the high-index material. This phenomenon is called total internal reflection and is the fundamental principle behind the operation of optical fibers.
Optical Fibers and Total Internal Reflection
Optical fibers consist of a high-refractive-index core surrounded by a low-refractive-index cladding layer. Light entering the fiber core through one end at the correct critical angle will bounce back whenever it reaches the boundary between the core and the cladding. This behavior effectively traps light inside the core, allowing the light pulses to propagate through the fiber with minimal loss over long distances.
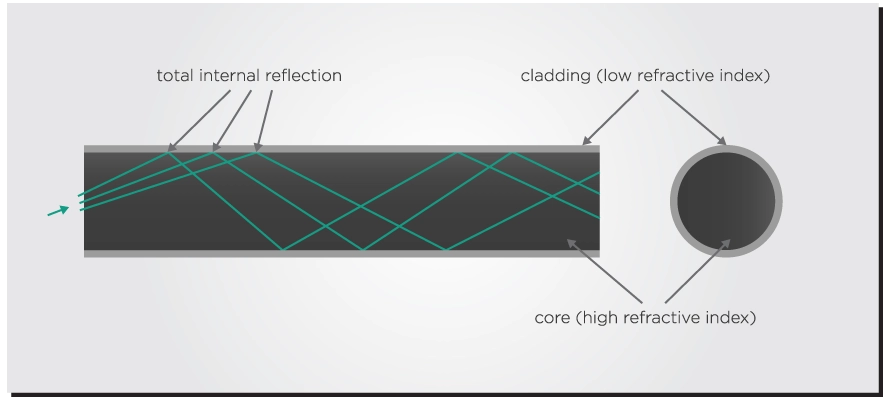
Optical fibers can achieve single-mode or multi-mode operation by carefully engineering the refractive indices of the core and cladding. Single-mode fibers have a small core diameter to transmit only a single optical mode or path. In contrast, multi-mode fibers have a larger core diameter, enabling the propagation of multiple modes simultaneously.
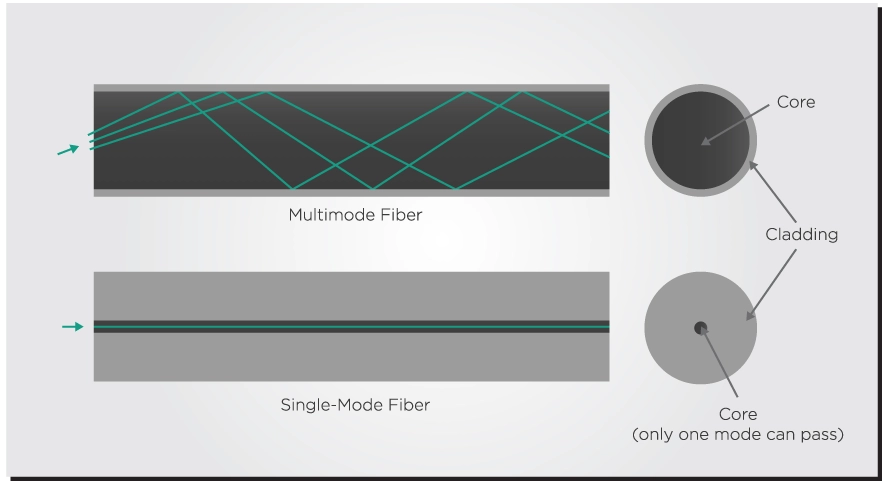
The quality of the light signal degrades when traveling through an optical fiber by a process called dispersion. The same phenomenon happens when a prism splits white light into several colors. The single-mode fiber design minimizes dispersion, making single-mode fibers ideal for long-distance communication. Since they are more susceptible to dispersion, multi-mode fibers are well-suited for short-distance applications such as local area networks (LANs).
The Role of Optical Transceivers in Fiber
Optical transceivers play a crucial role in fiber communications by converting electrical signals into optical signals for fiber transmission and vice versa when the optical signal is received. They act as the interface between fiber optical networks and electronic computing devices such as computers, routers, and switches.
As the name implies, an optical transceiver contains both a transmitter and a receiver within a single module. The transmitter has a laser or LED that generates light pulses representing the electronic data signal. On the receiving end, the receiver detects the optical signals and converts them back into electrical signals, which electronic devices can further process.
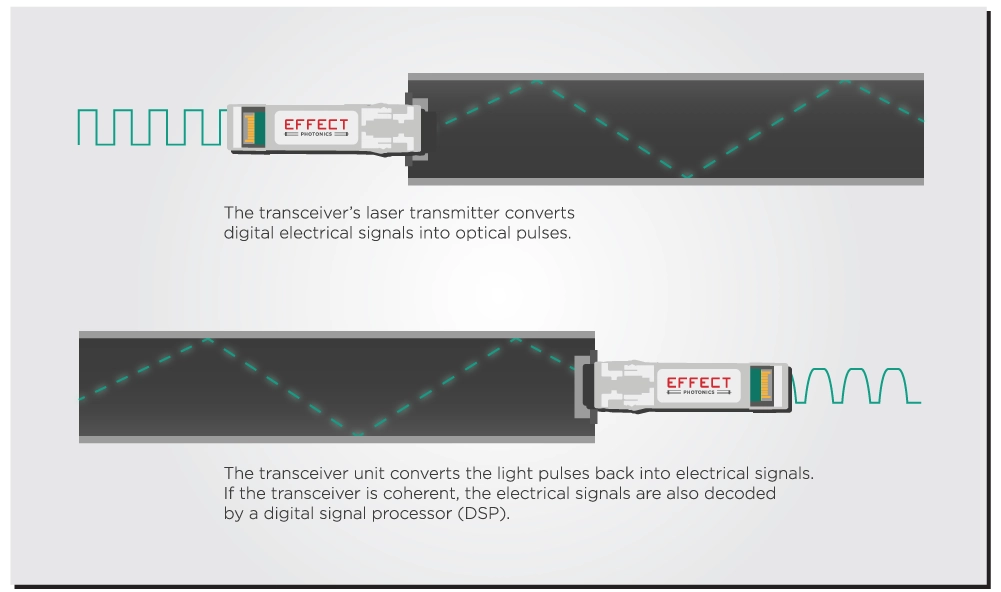
There are many different approaches to encode electrical data into light pulses. Two key approaches are intensity modulation/direct detection (IM-DD) and coherent transmission. IM-DD transmission only uses the amplitude of the light signal to encode data, while coherent transmission manipulates three different light properties to encode data: amplitude, phase, and polarization. These additional degrees of modulation allow for faster optical signals without compromising the transmission distance.
Takeaways
Optical fibers have transformed telecommunications in the last 50 years, enabling the rapid and efficient transmission of data over vast distances. By exploiting the principles of total internal reflection, these slender strands of glass or plastic carry pulses of light with minimal loss, ensuring high-speed communication worldwide. Optical transceivers act as the vital link between optical fiber and electronic networking devices, facilitating the conversion of electrical signals to optical signals and vice versa. Optical fibers and transceivers are at the forefront of our interconnected world, serving as the highways of light the digital age drives on.